7.3: Synapses
- Page ID
- 9578
\( \newcommand{\vecs}[1]{\overset { \scriptstyle \rightharpoonup} {\mathbf{#1}} } \)
\( \newcommand{\vecd}[1]{\overset{-\!-\!\rightharpoonup}{\vphantom{a}\smash {#1}}} \)
\( \newcommand{\id}{\mathrm{id}}\) \( \newcommand{\Span}{\mathrm{span}}\)
( \newcommand{\kernel}{\mathrm{null}\,}\) \( \newcommand{\range}{\mathrm{range}\,}\)
\( \newcommand{\RealPart}{\mathrm{Re}}\) \( \newcommand{\ImaginaryPart}{\mathrm{Im}}\)
\( \newcommand{\Argument}{\mathrm{Arg}}\) \( \newcommand{\norm}[1]{\| #1 \|}\)
\( \newcommand{\inner}[2]{\langle #1, #2 \rangle}\)
\( \newcommand{\Span}{\mathrm{span}}\)
\( \newcommand{\id}{\mathrm{id}}\)
\( \newcommand{\Span}{\mathrm{span}}\)
\( \newcommand{\kernel}{\mathrm{null}\,}\)
\( \newcommand{\range}{\mathrm{range}\,}\)
\( \newcommand{\RealPart}{\mathrm{Re}}\)
\( \newcommand{\ImaginaryPart}{\mathrm{Im}}\)
\( \newcommand{\Argument}{\mathrm{Arg}}\)
\( \newcommand{\norm}[1]{\| #1 \|}\)
\( \newcommand{\inner}[2]{\langle #1, #2 \rangle}\)
\( \newcommand{\Span}{\mathrm{span}}\) \( \newcommand{\AA}{\unicode[.8,0]{x212B}}\)
\( \newcommand{\vectorA}[1]{\vec{#1}} % arrow\)
\( \newcommand{\vectorAt}[1]{\vec{\text{#1}}} % arrow\)
\( \newcommand{\vectorB}[1]{\overset { \scriptstyle \rightharpoonup} {\mathbf{#1}} } \)
\( \newcommand{\vectorC}[1]{\textbf{#1}} \)
\( \newcommand{\vectorD}[1]{\overrightarrow{#1}} \)
\( \newcommand{\vectorDt}[1]{\overrightarrow{\text{#1}}} \)
\( \newcommand{\vectE}[1]{\overset{-\!-\!\rightharpoonup}{\vphantom{a}\smash{\mathbf {#1}}}} \)
\( \newcommand{\vecs}[1]{\overset { \scriptstyle \rightharpoonup} {\mathbf{#1}} } \)
\( \newcommand{\vecd}[1]{\overset{-\!-\!\rightharpoonup}{\vphantom{a}\smash {#1}}} \)
\(\newcommand{\avec}{\mathbf a}\) \(\newcommand{\bvec}{\mathbf b}\) \(\newcommand{\cvec}{\mathbf c}\) \(\newcommand{\dvec}{\mathbf d}\) \(\newcommand{\dtil}{\widetilde{\mathbf d}}\) \(\newcommand{\evec}{\mathbf e}\) \(\newcommand{\fvec}{\mathbf f}\) \(\newcommand{\nvec}{\mathbf n}\) \(\newcommand{\pvec}{\mathbf p}\) \(\newcommand{\qvec}{\mathbf q}\) \(\newcommand{\svec}{\mathbf s}\) \(\newcommand{\tvec}{\mathbf t}\) \(\newcommand{\uvec}{\mathbf u}\) \(\newcommand{\vvec}{\mathbf v}\) \(\newcommand{\wvec}{\mathbf w}\) \(\newcommand{\xvec}{\mathbf x}\) \(\newcommand{\yvec}{\mathbf y}\) \(\newcommand{\zvec}{\mathbf z}\) \(\newcommand{\rvec}{\mathbf r}\) \(\newcommand{\mvec}{\mathbf m}\) \(\newcommand{\zerovec}{\mathbf 0}\) \(\newcommand{\onevec}{\mathbf 1}\) \(\newcommand{\real}{\mathbb R}\) \(\newcommand{\twovec}[2]{\left[\begin{array}{r}#1 \\ #2 \end{array}\right]}\) \(\newcommand{\ctwovec}[2]{\left[\begin{array}{c}#1 \\ #2 \end{array}\right]}\) \(\newcommand{\threevec}[3]{\left[\begin{array}{r}#1 \\ #2 \\ #3 \end{array}\right]}\) \(\newcommand{\cthreevec}[3]{\left[\begin{array}{c}#1 \\ #2 \\ #3 \end{array}\right]}\) \(\newcommand{\fourvec}[4]{\left[\begin{array}{r}#1 \\ #2 \\ #3 \\ #4 \end{array}\right]}\) \(\newcommand{\cfourvec}[4]{\left[\begin{array}{c}#1 \\ #2 \\ #3 \\ #4 \end{array}\right]}\) \(\newcommand{\fivevec}[5]{\left[\begin{array}{r}#1 \\ #2 \\ #3 \\ #4 \\ #5 \\ \end{array}\right]}\) \(\newcommand{\cfivevec}[5]{\left[\begin{array}{c}#1 \\ #2 \\ #3 \\ #4 \\ #5 \\ \end{array}\right]}\) \(\newcommand{\mattwo}[4]{\left[\begin{array}{rr}#1 \amp #2 \\ #3 \amp #4 \\ \end{array}\right]}\) \(\newcommand{\laspan}[1]{\text{Span}\{#1\}}\) \(\newcommand{\bcal}{\cal B}\) \(\newcommand{\ccal}{\cal C}\) \(\newcommand{\scal}{\cal S}\) \(\newcommand{\wcal}{\cal W}\) \(\newcommand{\ecal}{\cal E}\) \(\newcommand{\coords}[2]{\left\{#1\right\}_{#2}}\) \(\newcommand{\gray}[1]{\color{gray}{#1}}\) \(\newcommand{\lgray}[1]{\color{lightgray}{#1}}\) \(\newcommand{\rank}{\operatorname{rank}}\) \(\newcommand{\row}{\text{Row}}\) \(\newcommand{\col}{\text{Col}}\) \(\renewcommand{\row}{\text{Row}}\) \(\newcommand{\nul}{\text{Nul}}\) \(\newcommand{\var}{\text{Var}}\) \(\newcommand{\corr}{\text{corr}}\) \(\newcommand{\len}[1]{\left|#1\right|}\) \(\newcommand{\bbar}{\overline{\bvec}}\) \(\newcommand{\bhat}{\widehat{\bvec}}\) \(\newcommand{\bperp}{\bvec^\perp}\) \(\newcommand{\xhat}{\widehat{\xvec}}\) \(\newcommand{\vhat}{\widehat{\vvec}}\) \(\newcommand{\uhat}{\widehat{\uvec}}\) \(\newcommand{\what}{\widehat{\wvec}}\) \(\newcommand{\Sighat}{\widehat{\Sigma}}\) \(\newcommand{\lt}{<}\) \(\newcommand{\gt}{>}\) \(\newcommand{\amp}{&}\) \(\definecolor{fillinmathshade}{gray}{0.9}\)- Explain how sample signals cross the synapse
Synaptic Transmission
The synapse or “gap” is the place where information is transmitted from one neuron to another. Synapses usually form between axon terminals and dendritic spines, but this is not universally true. There are also axon-to-axon, dendrite-to-dendrite, and axon-to-cell body synapses. The neuron transmitting the signal is called the presynaptic neuron, and the neuron receiving the signal is called the postsynaptic neuron. Note that these designations are relative to a particular synapse—most neurons are both presynaptic and postsynaptic. There are two types of synapses: chemical and electrical.
Chemical Synapses
When an action potential reaches the axon terminal it depolarizes the membrane and opens voltage-gated \(\ce{Na^{+}}\) channels. \(\ce{Na^{+}}\) ions enter the cell, further depolarizing the presynaptic membrane. This depolarization causes voltage-gated \(\ce{Ca^{3+}}\) channels to open. Calcium ions entering the cell initiate a signaling cascade that causes small membrane-bound vesicles, called synaptic vesicles, containing neurotransmitter molecules to fuse with the presynaptic membrane. Synaptic vesicles are shown in Figure 7.18, which is an image from a scanning electron microscope.
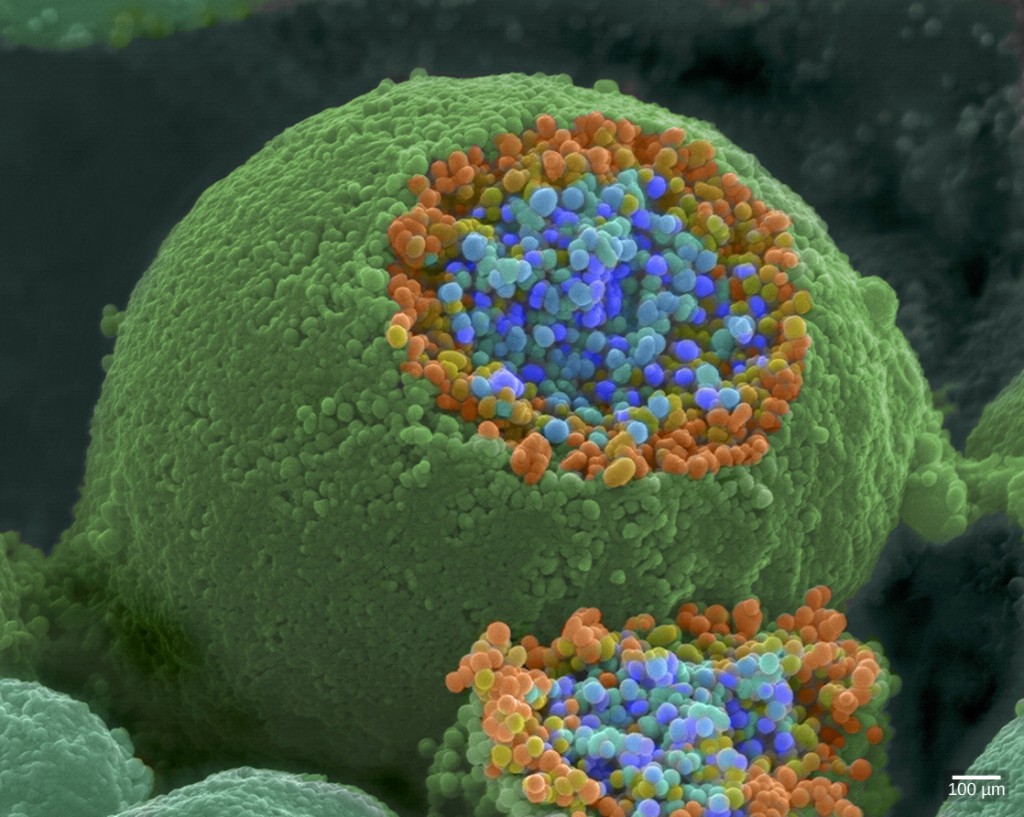
Fusion of a vesicle with the presynaptic membrane causes the neurotransmitter to be released into the synaptic cleft, the extracellular space between the presynaptic and postsynaptic membranes, as illustrated in Figure 7.19. The neurotransmitter diffuses across the synaptic cleft and binds to receptor proteins on the postsynaptic membrane.
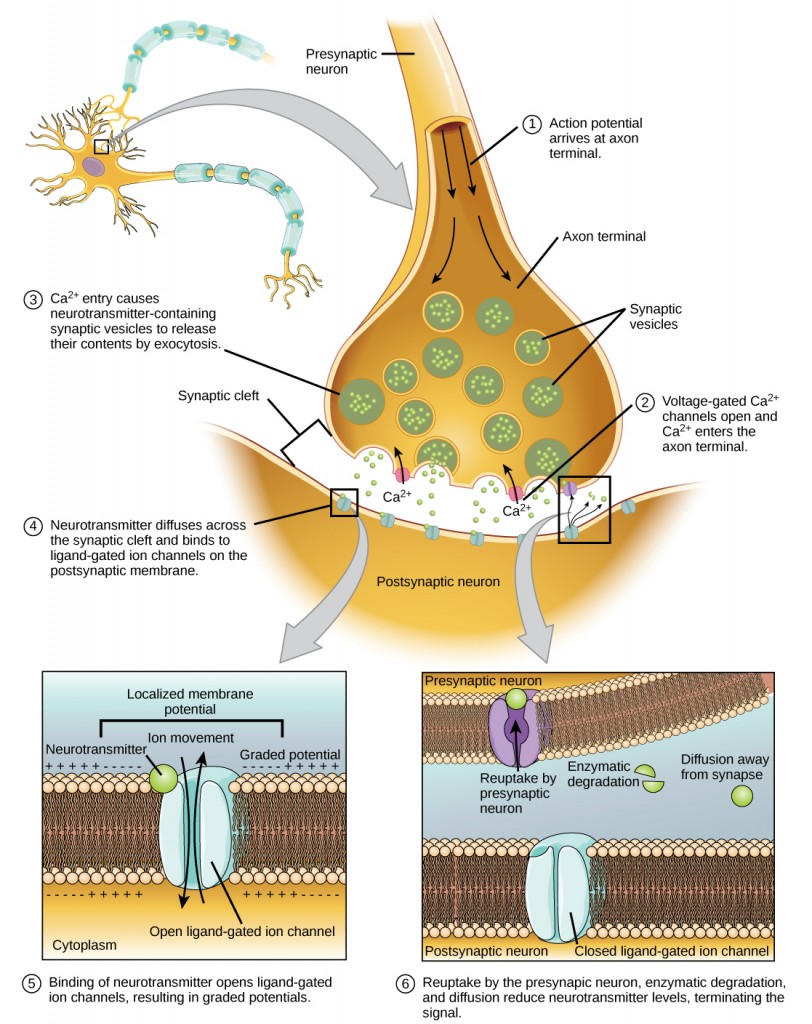
The binding of a specific neurotransmitter causes particular ion channels, in this case, ligand-gated channels, on the postsynaptic membrane to open. Neurotransmitters can either have excitatory or inhibitory effects on the postsynaptic membrane, as detailed in Table 7.2. For example, when acetylcholine is released at the synapse between a nerve and muscle (called the neuromuscular junction) by a presynaptic neuron, it causes postsynaptic Na+ channels to open. Na+ enters the postsynaptic cell and causes the postsynaptic membrane to depolarize. This depolarization is called an excitatory postsynaptic potential (EPSP) and makes the postsynaptic neuron more likely to fire an action potential. The release of neurotransmitter at inhibitory synapses causes inhibitory postsynaptic potentials (IPSPs), a hyperpolarization of the presynaptic membrane. For example, when the neurotransmitter GABA (gamma-aminobutyric acid) is released from a presynaptic neuron, it binds to and opens Cl– channels. Cl– ions enter the cell and hyperpolarizes the membrane, making the neuron less likely to fire an action potential.
Once neurotransmission has occurred, the neurotransmitter must be removed from the synaptic cleft so that the postsynaptic membrane can “reset” and be ready to receive another signal. This can be accomplished in three ways: the neurotransmitter can diffuse away from the synaptic cleft, it can be degraded by enzymes in the synaptic cleft, or it can be recycled (sometimes called reuptake) by the presynaptic neuron. Several drugs act at this step of neurotransmission. For example, some drugs that are given to Alzheimer’s patients work by inhibiting acetylcholinesterase, the enzyme that degrades acetylcholine. This inhibition of the enzyme essentially increases neurotransmission at synapses that release acetylcholine. Once released, the acetylcholine stays in the cleft and can continually bind and unbind to postsynaptic receptors.
Neurotransmitter | Example | Location |
---|---|---|
Acetylcholine | — | CNS and/or PNS |
Biogenic amine | Dopamine, serotonin, norepinephrine | CNS and/or PNS |
Amino acid | Glycine, glutamate, aspartate, gamma-aminobutyric acid | CNS |
Neuropeptide | Substance P, endorphins | CNS and/or PNS |
Design and/or act out events at the synapse! Here are some hints to help you design your review activity:
- You will need to think about how many peers you need to participate.
- Who will be which structure?
- How will your peers and you move with respect to each other?
- What is the overall goal of each movement you design (what is the function of each structure)?
Electrical Synapses
While electrical synapses are fewer in number than chemical synapses, they are found in all nervous systems and play important and unique roles. The mode of neurotransmission in electrical synapses is quite different from that in chemical synapses. In an electrical synapse, the presynaptic and postsynaptic membranes are very close together and are actually physically connected by channel proteins forming gap junctions. Gap junctions allow current to pass directly from one cell to the next. In addition to the ions that carry this current, other molecules, such as ATP, can diffuse through the large gap junction pores.
There are key differences between chemical and electrical synapses. Because chemical synapses depend on the release of neurotransmitter molecules from synaptic vesicles to pass on their signal, there is an approximately one-millisecond delay between when the axon potential reaches the presynaptic terminal and when the neurotransmitter leads to opening of postsynaptic ion channels. Additionally, this signaling is unidirectional. Signaling in electrical synapses, in contrast, is virtually instantaneous (which is important for synapses involved in key reflexes), and some electrical synapses are bidirectional. Electrical synapses are also more reliable as they are less likely to be blocked, and they are important for synchronizing the electrical activity of a group of neurons. For example, electrical synapses in the thalamus are thought to regulate slow-wave sleep, and disruption of these synapses can cause seizures.
Signal summation
Sometimes a single EPSP is strong enough to induce an action potential in the postsynaptic neuron, but often multiple presynaptic inputs must create EPSPs around the same time for the postsynaptic neuron to be sufficiently depolarized to fire an action potential. This process is called summation and occurs at the axon hillock, as illustrated in Figure 7.20. Additionally, one neuron often has inputs from many presynaptic neurons—some excitatory and some inhibitory—so IPSPs can cancel out EPSPs and vice versa. It is the net change in postsynaptic membrane voltage that determines whether the postsynaptic cell has reached its threshold of excitation needed to fire an action potential. Together, synaptic summation and the threshold for excitation act as a filter so that random “noise” in the system is not transmitted as important information.

Synaptic plasticity
Synapses are not static structures. They can be weakened or strengthened. They can be broken, and new synapses can be made. Synaptic plasticity allows for these changes, which are all needed for a functioning nervous system. In fact, synaptic plasticity is the basis of learning and memory. Two processes in particular, long-term potentiation (LTP) and long-term depression (LTD) are important forms of synaptic plasticity that occur in synapses in the hippocampus, a brain region that is involved in storing memories.
Long-term potentiation (LTP)
Long-term potentiation (LTP) is a persistent strengthening of a synaptic connection. LTP is based on the Hebbian principle: cells that fire together wire together. There are various mechanisms, none fully understood, behind the synaptic strengthening seen with LTP. One known mechanism involves a type of postsynaptic glutamate receptor, called NMDA (N-Methyl-D-aspartate) receptors, shown in Figure 7.21. These receptors are normally blocked by magnesium ions; however, when the postsynaptic neuron is depolarized by multiple presynaptic inputs in quick succession (either from one neuron or multiple neurons), the magnesium ions are forced out allowing Ca ions to pass into the postsynaptic cell. Next, Ca2+ions entering the cell initiate a signaling cascade that causes a different type of glutamate receptor, called AMPA (α-amino-3-hydroxy-5-methyl-4-isoxazolepropionic acid) receptors, to be inserted into the postsynaptic membrane, since activated AMPA receptors allow positive ions to enter the cell. So, the next time glutamate is released from the presynaptic membrane, it will have a larger excitatory effect (EPSP) on the postsynaptic cell because the binding of glutamate to these AMPA receptors will allow more positive ions into the cell. The insertion of additional AMPA receptors strengthens the synapse and means that the postsynaptic neuron is more likely to fire in response to presynaptic neurotransmitter release. Some drugs of abuse co-opt the LTP pathway, and this synaptic strengthening can lead to addiction.
Long-term depression (LTD)
Long-term depression (LTD) is essentially the reverse of LTP: it is a long-term weakening of a synaptic connection. One mechanism known to cause LTD also involves AMPA receptors. In this situation, calcium that enters through NMDA receptors initiates a different signaling cascade, which results in the removal of AMPA receptors from the postsynaptic membrane, as illustrated in Figure 7.21. The decrease in AMPA receptors in the membrane makes the postsynaptic neuron less responsive to glutamate released from the presynaptic neuron. While it may seem counterintuitive, LTD may be just as important for learning and memory as LTP. The weakening and pruning of unused synapses allow for unimportant connections to be lost and makes the synapses that have undergone LTP that much stronger by comparison.
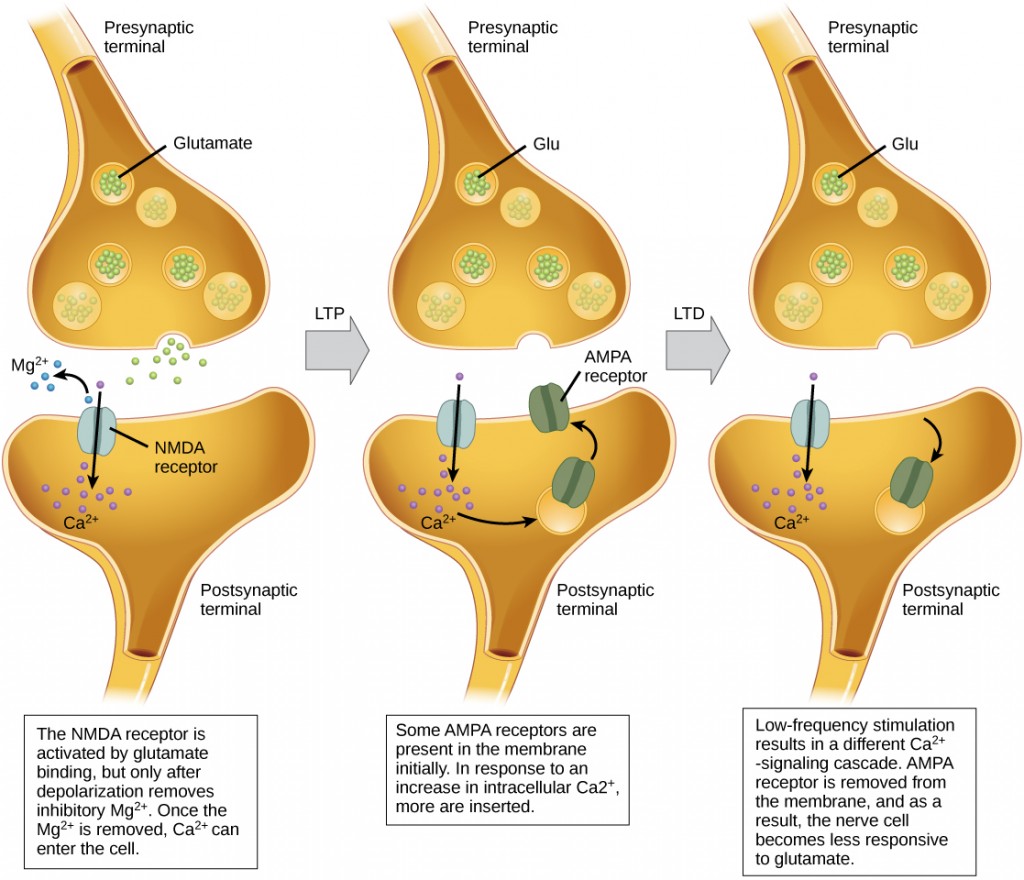
For a neuron to fire an action potential, its membrane must reach ________.
a. hyperpolarization
b. the threshold of excitation
c. the refractory period
d. inhibitory postsynaptic potentia
After an action potential, the opening of additional voltage-gated ________ channels and the inactivation of sodium channels, cause the membrane to return to its resting membrane potential.
a. sodium
b. potassium
c. calcium
d. chloride
What is the term for protein channels that connect two neurons at an electrical synapse?
a. synaptic vesicles
b. voltage-gated ion channels
c. gap junction protein
d. sodium-potassium exchange pumps
How does myelin aid propagation of an action potential along an axon? How do the nodes of Ranvier help this process?
What are the main steps in chemical neurotransmission?