5.11: Touch- Central Processing
- Page ID
- 69995
\( \newcommand{\vecs}[1]{\overset { \scriptstyle \rightharpoonup} {\mathbf{#1}} } \)
\( \newcommand{\vecd}[1]{\overset{-\!-\!\rightharpoonup}{\vphantom{a}\smash {#1}}} \)
\( \newcommand{\id}{\mathrm{id}}\) \( \newcommand{\Span}{\mathrm{span}}\)
( \newcommand{\kernel}{\mathrm{null}\,}\) \( \newcommand{\range}{\mathrm{range}\,}\)
\( \newcommand{\RealPart}{\mathrm{Re}}\) \( \newcommand{\ImaginaryPart}{\mathrm{Im}}\)
\( \newcommand{\Argument}{\mathrm{Arg}}\) \( \newcommand{\norm}[1]{\| #1 \|}\)
\( \newcommand{\inner}[2]{\langle #1, #2 \rangle}\)
\( \newcommand{\Span}{\mathrm{span}}\)
\( \newcommand{\id}{\mathrm{id}}\)
\( \newcommand{\Span}{\mathrm{span}}\)
\( \newcommand{\kernel}{\mathrm{null}\,}\)
\( \newcommand{\range}{\mathrm{range}\,}\)
\( \newcommand{\RealPart}{\mathrm{Re}}\)
\( \newcommand{\ImaginaryPart}{\mathrm{Im}}\)
\( \newcommand{\Argument}{\mathrm{Arg}}\)
\( \newcommand{\norm}[1]{\| #1 \|}\)
\( \newcommand{\inner}[2]{\langle #1, #2 \rangle}\)
\( \newcommand{\Span}{\mathrm{span}}\) \( \newcommand{\AA}{\unicode[.8,0]{x212B}}\)
\( \newcommand{\vectorA}[1]{\vec{#1}} % arrow\)
\( \newcommand{\vectorAt}[1]{\vec{\text{#1}}} % arrow\)
\( \newcommand{\vectorB}[1]{\overset { \scriptstyle \rightharpoonup} {\mathbf{#1}} } \)
\( \newcommand{\vectorC}[1]{\textbf{#1}} \)
\( \newcommand{\vectorD}[1]{\overrightarrow{#1}} \)
\( \newcommand{\vectorDt}[1]{\overrightarrow{\text{#1}}} \)
\( \newcommand{\vectE}[1]{\overset{-\!-\!\rightharpoonup}{\vphantom{a}\smash{\mathbf {#1}}}} \)
\( \newcommand{\vecs}[1]{\overset { \scriptstyle \rightharpoonup} {\mathbf{#1}} } \)
\( \newcommand{\vecd}[1]{\overset{-\!-\!\rightharpoonup}{\vphantom{a}\smash {#1}}} \)
\(\newcommand{\avec}{\mathbf a}\) \(\newcommand{\bvec}{\mathbf b}\) \(\newcommand{\cvec}{\mathbf c}\) \(\newcommand{\dvec}{\mathbf d}\) \(\newcommand{\dtil}{\widetilde{\mathbf d}}\) \(\newcommand{\evec}{\mathbf e}\) \(\newcommand{\fvec}{\mathbf f}\) \(\newcommand{\nvec}{\mathbf n}\) \(\newcommand{\pvec}{\mathbf p}\) \(\newcommand{\qvec}{\mathbf q}\) \(\newcommand{\svec}{\mathbf s}\) \(\newcommand{\tvec}{\mathbf t}\) \(\newcommand{\uvec}{\mathbf u}\) \(\newcommand{\vvec}{\mathbf v}\) \(\newcommand{\wvec}{\mathbf w}\) \(\newcommand{\xvec}{\mathbf x}\) \(\newcommand{\yvec}{\mathbf y}\) \(\newcommand{\zvec}{\mathbf z}\) \(\newcommand{\rvec}{\mathbf r}\) \(\newcommand{\mvec}{\mathbf m}\) \(\newcommand{\zerovec}{\mathbf 0}\) \(\newcommand{\onevec}{\mathbf 1}\) \(\newcommand{\real}{\mathbb R}\) \(\newcommand{\twovec}[2]{\left[\begin{array}{r}#1 \\ #2 \end{array}\right]}\) \(\newcommand{\ctwovec}[2]{\left[\begin{array}{c}#1 \\ #2 \end{array}\right]}\) \(\newcommand{\threevec}[3]{\left[\begin{array}{r}#1 \\ #2 \\ #3 \end{array}\right]}\) \(\newcommand{\cthreevec}[3]{\left[\begin{array}{c}#1 \\ #2 \\ #3 \end{array}\right]}\) \(\newcommand{\fourvec}[4]{\left[\begin{array}{r}#1 \\ #2 \\ #3 \\ #4 \end{array}\right]}\) \(\newcommand{\cfourvec}[4]{\left[\begin{array}{c}#1 \\ #2 \\ #3 \\ #4 \end{array}\right]}\) \(\newcommand{\fivevec}[5]{\left[\begin{array}{r}#1 \\ #2 \\ #3 \\ #4 \\ #5 \\ \end{array}\right]}\) \(\newcommand{\cfivevec}[5]{\left[\begin{array}{c}#1 \\ #2 \\ #3 \\ #4 \\ #5 \\ \end{array}\right]}\) \(\newcommand{\mattwo}[4]{\left[\begin{array}{rr}#1 \amp #2 \\ #3 \amp #4 \\ \end{array}\right]}\) \(\newcommand{\laspan}[1]{\text{Span}\{#1\}}\) \(\newcommand{\bcal}{\cal B}\) \(\newcommand{\ccal}{\cal C}\) \(\newcommand{\scal}{\cal S}\) \(\newcommand{\wcal}{\cal W}\) \(\newcommand{\ecal}{\cal E}\) \(\newcommand{\coords}[2]{\left\{#1\right\}_{#2}}\) \(\newcommand{\gray}[1]{\color{gray}{#1}}\) \(\newcommand{\lgray}[1]{\color{lightgray}{#1}}\) \(\newcommand{\rank}{\operatorname{rank}}\) \(\newcommand{\row}{\text{Row}}\) \(\newcommand{\col}{\text{Col}}\) \(\renewcommand{\row}{\text{Row}}\) \(\newcommand{\nul}{\text{Nul}}\) \(\newcommand{\var}{\text{Var}}\) \(\newcommand{\corr}{\text{corr}}\) \(\newcommand{\len}[1]{\left|#1\right|}\) \(\newcommand{\bbar}{\overline{\bvec}}\) \(\newcommand{\bhat}{\widehat{\bvec}}\) \(\newcommand{\bperp}{\bvec^\perp}\) \(\newcommand{\xhat}{\widehat{\xvec}}\) \(\newcommand{\vhat}{\widehat{\vvec}}\) \(\newcommand{\uhat}{\widehat{\uvec}}\) \(\newcommand{\what}{\widehat{\wvec}}\) \(\newcommand{\Sighat}{\widehat{\Sigma}}\) \(\newcommand{\lt}{<}\) \(\newcommand{\gt}{>}\) \(\newcommand{\amp}{&}\) \(\definecolor{fillinmathshade}{gray}{0.9}\)- Glossary terms
- Scientist links to learn more!
- Key Takeaways
- Test Yourself
- Additional Review
Receptive Fields and Lateral Inhibition
The receptive fields of the sensory neurons become more complex as information moves up the pathway. We saw in the last lesson that mechanoreceptors have receptive fields that, when touched, activate the neuron. The mechanoreceptors synapse on neurons in the dorsal column, and those neurons have more complex receptive fields. The dorsal column nuclei have receptive fields that are divided into center and surround regions. The center of the receptive field is a result of direct innervation from the mechanoreceptors. If a stimulus touches the skin in the center of a dorsal column neuron’s receptive field, the neuron will increase its firing rate. The center / surround structure is like that of bipolar and ganglion cells in the vision system.

The surround region of the receptive field is a result of indirect communication between the receptor neurons and the dorsal column neurons via inhibitory interneurons. The surround has an inhibitory effect on the dorsal column neuron. If a stimulus touches the skin in the surround of a dorsal column neuron’s receptive field, the neuron will decrease its firing rate.

Lateral Inhibition
The center-surround structure of the receptive field is critical for lateral inhibition to occur. Lateral inhibition is the ability of the sensory systems to enhance the perception of edges of stimuli. At a point or an edge of a stimulus, because of the inhibitory interneurons, the perceived stimulus strength will be enhanced compared to the actual stimulus strength.

Pathway to Brain
Dorsal Column-Medical Lemniscus Pathway
The pathway from the periphery to the somatosensory cortex consists of three neurons: a first-order sensory neuron, a second-order medulla neuron, and a third-order thalamic neuron.
Specifically, the primary afferent sensory fibers (sensory neurons) have their cell bodies located in the dorsal root ganglion, a structure that lies just outside of the spinal cord. The axons of these first-order neurons enter the ipsilateral dorsal side of the spinal cord. Some axon collaterals terminate in the spinal cord and are important for reflexes.
The main axon branch ascends the spinal cord toward the brain, via the dorsal column, synapsing in the dorsal column nuclei located in the medulla of the brainstem. The axons of sensory neurons in the lower body remain separate from the axons of sensory neurons in the upper body throughout the pathway. These two populations of neurons synapse in different regions of the medulla. The lower body axons synapse in the gracile nucleus, whereas the upper body axons synapse in cuneate nucleus. The second-order neurons within the medulla have axons that cross the midline, or decussate, and ascend via a white matter tract called the medial lemniscus.
The medulla axons synapse in the ventral posterior lateral nucleus of the thalamus. The third-order thalamic neurons then project to the primary somatosensory cortex located in the postcentral gyrus in the parietal lobe.

View the thalamus using the BrainFacts.org 3D Brain
View the primary somatosensory cortex using the BrainFacts.org 3D Brain
Trigeminal Pathway
The pathway from the face and head to the somatosensory cortex also consists of three neurons. Sensory receptors in the face and head send information to the brain via cranial nerve V, the trigeminal nerve. The first-order neurons have their cell bodies in the trigeminal ganglion, located just outside of the brainstem, and they project to the ipsilateral trigeminal nucleus in the pons. The second-order neurons within the pons cross the midline and project up to the ventral posterior medial nucleus of the thalamus. These third-order thalamic neurons then send projections to the face region of the somatosensory cortex.


Primary Somatosensory Cortex
Anatomy
The primary somatosensory cortex is the postcentral gyrus located in the parietal lobe. It is divided into four regions, each with its own input and function: areas 3a, 3b, 1, and 2. The neurons within these regions are arranged in columns, similar to other areas of the cortex.
Most touch information from mechanoreceptors inputs to region 3b, whereas most proprioceptive information from the muscles inputs to region 3a. These regions then send and receive information from areas 1 and 2. As processing of somatosensory information continues, the stimuli required to activate neurons becomes more complex. For example, area 1 is involved in sensing texture, and area 2 is involved in sensing size and shape of an object. The posterior parietal cortex, an important output region of the somatosensory cortex, lies caudal to the postcentral gyrus; areas 5 and 7 are downstream structures that continue to process touch.

Somatotopic Map
The receptive fields of each higher order neuron increases in size and complexity, but even cortical neurons are associated with a specific region of the body. The mapping of this organization was discovered during brain surgery. In epilepsy surgery, parts of the operation are performed as the person is awake. The famed neurosurgeon Wilder Penfield electrically stimulated a small section of the primary somatosensory cortex (S1), then asked the person what they felt. Patients would respond that they feel sensations in their hand when the medial S1 was activated, the little finger when a more ventral area was activated, and so on. In repeating this process while moving slowly across the span of S1, Penfield mapped out the organization of S1. He discovered that the sensory and motor cortices are organized by the region of the body they represent, so neurons that respond to sensation in the fingers are located close to the neurons that respond to sensation in the hand.
One key observation in doing this process was the volume of brain regions corresponding to different body parts was not necessarily proportional to the volume of skin: for example, a large portion of the cortex was dedicated to sensory information from the lips or the fingertips, while very little was dedicated to the shoulders or trunk. This observation indicates that certain areas of the body are more densely packed (lips, fingertips) with sensory neurons compared to others (shoulders, trunk). Regions with high receptor density in the skin, and, therefore, fine two-point discrimination, have more cortical space devoted to them. This means that the cortical representation of the body is not true to actual physical proportions.
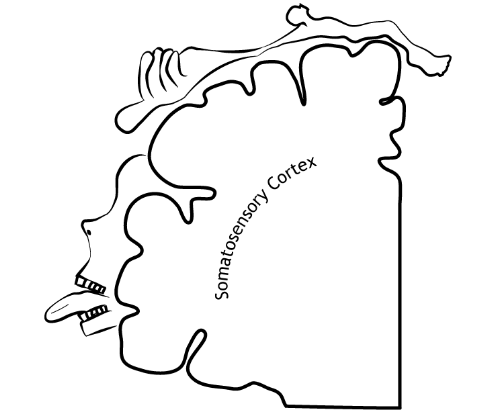
A homunculus is a cartoon representation of what a body would look like if actual body size was proportional to the cortical representation. The hands and lips would be excessively large while the torso, arms, and legs, would be relatively small. Importantly, most homunculus representations are based on the mapping of male brains, but far less research has been done on the mapping of female brains.

Higher-Level Processing of Touch Information
The primary somatosensory cortex sends projections to other parietal lobe regions for higher-level processing of touch information.
Secondary Somatosensory Cortex
The secondary somatosensory cortex (SII) is located in the inferior parietal lobe, just above the lateral fissure. This region, like the dorsal stream of visual processing, is responsible for object recognition, discerning texture, shape, and size. The SII also has receptive fields that represent bilateral regions of the body, so both hemispheres will be activated by touch on either side of the body. The SII sends projections to the posterior parietal cortex, the premotor cortex, the amygdala, and the hippocampus.
Posterior Parietal Cortex
The posterior parietal cortex recognizes touch characteristics like orientation and movement. It is also important for combining the touch and motor components of actions like grasping. The posterior parietal cortex outputs to the frontal motor cortex.

View the posterior parietal cortex using the BrainFacts.org 3D Brain
Cortical Plasticity
In adulthood, the brain is plastic, meaning synaptic connections can rearrange under certain conditions. The somatosensory cortex has been studied with regard to its plasticity. Experience-based plasticity was initially proposed by Donald Hebb and is often referred to as Hebbian plasticity. This theory postulates that experience can enhance or reduce the activity of synapses to ultimately alter their function. When a presynaptic neuron repeatedly signals to a postsynaptic neuron, the synapse will undergo plasticity, changing structure to stabilize and strengthen the function of the synapse. Simplistically, the phrase “neurons that fire together, wire together” is often used to describe Hebbian plasticity. Plastic changes can include altering the amount of neurotransmitter release or altering the number of postsynaptic receptors.
Multiple principles of experience-dependent plasticity have been identified to build off Hebb’s original idea. We will focus on five of those principles below.
- Use it or lose it. The idea behind this principle is that neural connections that are used consistently are maintained and strengthened. When a synapse is strengthened, it will produce stronger EPSPs that bring the cell membrane potential closer to threshold potential. If neural connections are not used consistently, then those synapses are removed, or lost.
- Use it to improve it. The idea behind this principle is that connections that are consistently used continue to improve in function.
- Repetition of stimulation. Synaptic strength can be enhanced by repeated stimulation.
- Intensity of stimulation. The intensity of the stimulation will alter synaptic strength.
- Regional and functional specificity. Experience-dependent plasticity is specific to the region and function that is receiving the experience. This is to say, providing specific experience to one region of the brain will enhance plasticity within that one region, and will not globally enhance plasticity across the entire brain.
Measuring Cortical Plasticity
Michael Merzenich is a researcher that has enlightened our understanding of cortical plasticity within the somatosensory system. He used microelectrodes to stimulate and record from the somatosensory cortex to create detailed cortical maps, similar to the homunculus that we discussed earlier. Dr. Merzenich completed a number of experiments that demonstrated principles of experience-dependent plasticity, some of which are highlighted below.
In one of his experiment, he demonstrated that amputation or loss of a finger, for example, will lead to the associated cortical space to be functionally remapped by input from neighboring regions of the hand. The cortical neurons do not die, they begin to be activated by a different region of the body.

An additional experiment showed that when two adjacent fingers were sewn together so that they moved as a unit, a new cortical map developed that was specific to the merged fingers. Thus, cortical maps can be removed or created anew.

Likewise, cortical representation can expand with use or practice. Repeated training of certain fingers can lead to an increase in cortical space mapped to those digits.
Key Takeaways
- Receptive fields become more complex as information moves through the touch pathway
- Lateral inhibition enhances edges and borders by affecting the perceived stimulus strength
- Mechanoreceptor afferents synapse in the dorsal column nuclei in the medulla. Information then decussates and synapses in the ventral posterior nucleus of the thalamus before traveling to the primary somatosensory cortex
- Sensory axons from the lower body synapses in the gracile nucleus in the dorsal column
- Sensory axons from the upper body synapses in the cuneate nucleus in the dorsal column
- Information from the neck and body synapse in the ventral posterior lateral nucleus of the thalamus
- Information from the head and face synapse in the ventral posterior medial nucleus of the thalamus
- The primary somatosensory cortex is organized in a somatotopic map
- The cortex is plastic and connections can change with experience
Test Yourself!
Additional Review
- What is the anatomical name of the primary somatosensory cortex?
- After somatosensory information leaves the brainstem, it must relay through which structure before reaching the primary somatosensory cortex?
Attributions
Portions of this chapter were remixed and revised from the following sources:
- Foundations of Neuroscience by Casey Henley. The original work is licensed under a Creative Commons Attribution-NonCommercial-ShareAlike 4.0 International License
- Open Neuroscience Initiative by Austin Lim. The original work is licensed under a Creative Commons Attribution-NonCommercial 4.0 International License.
Media Attributions
- TouchReceptiveFieldCenter © Casey Henley is licensed under a CC BY-NC-SA (Attribution NonCommercial ShareAlike) license
- Touch Receptive Field Surround © Casey Henley is licensed under a CC BY-NC-SA (Attribution NonCommercial ShareAlike) license
- Touch Lateral Inhibition © Casey Henley is licensed under a CC BY-NC-SA (Attribution NonCommercial ShareAlike) license
- Touch Pathway From Body © Casey Henley is licensed under a CC BY-NC-SA (Attribution NonCommercial ShareAlike) license
- Touch Pathway From Face © Casey Henley is licensed under a CC BY-NC-SA (Attribution NonCommercial ShareAlike) license
- Touch Pathways © Casey Henley is licensed under a CC BY-NC-SA (Attribution NonCommercial ShareAlike) license
- Postcentral Gyrus © Casey Henley is licensed under a CC BY-NC-SA (Attribution NonCommercial ShareAlike) license
- Somatotopic Map © Casey Henley is licensed under a CC BY-NC-SA (Attribution NonCommercial ShareAlike) license
- Sensory Homonculus © Mpj29 adapted by Valerie Hedges is licensed under a CC BY-SA (Attribution ShareAlike) license
- Somatosensory Streams © Casey Henley is licensed under a CC BY-NC-SA (Attribution NonCommercial ShareAlike) license
- CorticalPlasticity © Casey Henley is licensed under a CC BY-NC-SA (Attribution NonCommercial ShareAlike) license
- Digit merge © Valerie Hedges is licensed under a CC BY-NC-SA (Attribution NonCommercial ShareAlike) license