1.8: Tooth Development
- Page ID
- 41544
\( \newcommand{\vecs}[1]{\overset { \scriptstyle \rightharpoonup} {\mathbf{#1}} } \)
\( \newcommand{\vecd}[1]{\overset{-\!-\!\rightharpoonup}{\vphantom{a}\smash {#1}}} \)
\( \newcommand{\id}{\mathrm{id}}\) \( \newcommand{\Span}{\mathrm{span}}\)
( \newcommand{\kernel}{\mathrm{null}\,}\) \( \newcommand{\range}{\mathrm{range}\,}\)
\( \newcommand{\RealPart}{\mathrm{Re}}\) \( \newcommand{\ImaginaryPart}{\mathrm{Im}}\)
\( \newcommand{\Argument}{\mathrm{Arg}}\) \( \newcommand{\norm}[1]{\| #1 \|}\)
\( \newcommand{\inner}[2]{\langle #1, #2 \rangle}\)
\( \newcommand{\Span}{\mathrm{span}}\)
\( \newcommand{\id}{\mathrm{id}}\)
\( \newcommand{\Span}{\mathrm{span}}\)
\( \newcommand{\kernel}{\mathrm{null}\,}\)
\( \newcommand{\range}{\mathrm{range}\,}\)
\( \newcommand{\RealPart}{\mathrm{Re}}\)
\( \newcommand{\ImaginaryPart}{\mathrm{Im}}\)
\( \newcommand{\Argument}{\mathrm{Arg}}\)
\( \newcommand{\norm}[1]{\| #1 \|}\)
\( \newcommand{\inner}[2]{\langle #1, #2 \rangle}\)
\( \newcommand{\Span}{\mathrm{span}}\) \( \newcommand{\AA}{\unicode[.8,0]{x212B}}\)
\( \newcommand{\vectorA}[1]{\vec{#1}} % arrow\)
\( \newcommand{\vectorAt}[1]{\vec{\text{#1}}} % arrow\)
\( \newcommand{\vectorB}[1]{\overset { \scriptstyle \rightharpoonup} {\mathbf{#1}} } \)
\( \newcommand{\vectorC}[1]{\textbf{#1}} \)
\( \newcommand{\vectorD}[1]{\overrightarrow{#1}} \)
\( \newcommand{\vectorDt}[1]{\overrightarrow{\text{#1}}} \)
\( \newcommand{\vectE}[1]{\overset{-\!-\!\rightharpoonup}{\vphantom{a}\smash{\mathbf {#1}}}} \)
\( \newcommand{\vecs}[1]{\overset { \scriptstyle \rightharpoonup} {\mathbf{#1}} } \)
\( \newcommand{\vecd}[1]{\overset{-\!-\!\rightharpoonup}{\vphantom{a}\smash {#1}}} \)
\(\newcommand{\avec}{\mathbf a}\) \(\newcommand{\bvec}{\mathbf b}\) \(\newcommand{\cvec}{\mathbf c}\) \(\newcommand{\dvec}{\mathbf d}\) \(\newcommand{\dtil}{\widetilde{\mathbf d}}\) \(\newcommand{\evec}{\mathbf e}\) \(\newcommand{\fvec}{\mathbf f}\) \(\newcommand{\nvec}{\mathbf n}\) \(\newcommand{\pvec}{\mathbf p}\) \(\newcommand{\qvec}{\mathbf q}\) \(\newcommand{\svec}{\mathbf s}\) \(\newcommand{\tvec}{\mathbf t}\) \(\newcommand{\uvec}{\mathbf u}\) \(\newcommand{\vvec}{\mathbf v}\) \(\newcommand{\wvec}{\mathbf w}\) \(\newcommand{\xvec}{\mathbf x}\) \(\newcommand{\yvec}{\mathbf y}\) \(\newcommand{\zvec}{\mathbf z}\) \(\newcommand{\rvec}{\mathbf r}\) \(\newcommand{\mvec}{\mathbf m}\) \(\newcommand{\zerovec}{\mathbf 0}\) \(\newcommand{\onevec}{\mathbf 1}\) \(\newcommand{\real}{\mathbb R}\) \(\newcommand{\twovec}[2]{\left[\begin{array}{r}#1 \\ #2 \end{array}\right]}\) \(\newcommand{\ctwovec}[2]{\left[\begin{array}{c}#1 \\ #2 \end{array}\right]}\) \(\newcommand{\threevec}[3]{\left[\begin{array}{r}#1 \\ #2 \\ #3 \end{array}\right]}\) \(\newcommand{\cthreevec}[3]{\left[\begin{array}{c}#1 \\ #2 \\ #3 \end{array}\right]}\) \(\newcommand{\fourvec}[4]{\left[\begin{array}{r}#1 \\ #2 \\ #3 \\ #4 \end{array}\right]}\) \(\newcommand{\cfourvec}[4]{\left[\begin{array}{c}#1 \\ #2 \\ #3 \\ #4 \end{array}\right]}\) \(\newcommand{\fivevec}[5]{\left[\begin{array}{r}#1 \\ #2 \\ #3 \\ #4 \\ #5 \\ \end{array}\right]}\) \(\newcommand{\cfivevec}[5]{\left[\begin{array}{c}#1 \\ #2 \\ #3 \\ #4 \\ #5 \\ \end{array}\right]}\) \(\newcommand{\mattwo}[4]{\left[\begin{array}{rr}#1 \amp #2 \\ #3 \amp #4 \\ \end{array}\right]}\) \(\newcommand{\laspan}[1]{\text{Span}\{#1\}}\) \(\newcommand{\bcal}{\cal B}\) \(\newcommand{\ccal}{\cal C}\) \(\newcommand{\scal}{\cal S}\) \(\newcommand{\wcal}{\cal W}\) \(\newcommand{\ecal}{\cal E}\) \(\newcommand{\coords}[2]{\left\{#1\right\}_{#2}}\) \(\newcommand{\gray}[1]{\color{gray}{#1}}\) \(\newcommand{\lgray}[1]{\color{lightgray}{#1}}\) \(\newcommand{\rank}{\operatorname{rank}}\) \(\newcommand{\row}{\text{Row}}\) \(\newcommand{\col}{\text{Col}}\) \(\renewcommand{\row}{\text{Row}}\) \(\newcommand{\nul}{\text{Nul}}\) \(\newcommand{\var}{\text{Var}}\) \(\newcommand{\corr}{\text{corr}}\) \(\newcommand{\len}[1]{\left|#1\right|}\) \(\newcommand{\bbar}{\overline{\bvec}}\) \(\newcommand{\bhat}{\widehat{\bvec}}\) \(\newcommand{\bperp}{\bvec^\perp}\) \(\newcommand{\xhat}{\widehat{\xvec}}\) \(\newcommand{\vhat}{\widehat{\vvec}}\) \(\newcommand{\uhat}{\widehat{\uvec}}\) \(\newcommand{\what}{\widehat{\wvec}}\) \(\newcommand{\Sighat}{\widehat{\Sigma}}\) \(\newcommand{\lt}{<}\) \(\newcommand{\gt}{>}\) \(\newcommand{\amp}{&}\) \(\definecolor{fillinmathshade}{gray}{0.9}\)- Overview
- Tooth development
- Initiation stage
- Bud stage
- Cap stage
- Bell stage
- Crown stage
- Root development
- Root dentin formation
- Cementum & pulp formation
- Multirooted teeth
- Periodontal ligament and alveolar bone
- Tooth eruption
- Clinical application
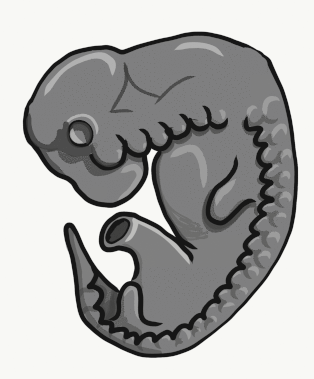
Overview of tooth formation
In this chapter we cover major concepts in tooth development, saving many of the details for subsequent chapters. The first big concept is timing. Both the primary and succedaneous teeth begin developing early in embryonic development—around weeks 5 or 6. To put this in perspective, that is before the mouth forms– the pharynx is still separated from the outside by the oropharyngeal membrane. The process of tooth development begins around weeks 5 or 6, when it finishes is complex. The crown develops during the embryonic period, the roots develop after birth, and the PDL and junctional epithelium form during tooth eruption.
Teeth develop is a fashion similar to hair follicles, they arise from invaginations of epithelium, derived from ectoderm. Instead of being called a tooth follicle, teeth grow from tooth buds. Like hair follicles, newer teeth erupt after older teeth are exfoliated (shed). Unlike hair follicles, tooth replacement only happens once (making the succedaneous teeth “permanent”).
Some teeth form before others, but the process of tooth formation is similar for all teeth. First, epithelial cells are induced to form a tooth bud. This happens because of the secretion of morphogens from neural crest cells below. You can’t see this under the microscope (not without more specialized tools than the H&E stain). As a result, this phase is often glossed over in histology books. That is a shame, because getting the correct number of teeth to form requires complex communication. Not only do the correct number need to form, their spacing must be determined at this time as well. There are more names for later events (usually named after long dead European men), and those names appear on license exams, so we cover those in detail as well. Lucky you.
Before going into detail, though, lets cover big concepts related to formation of the teeth. First, regions of epithelial cells proliferate and invaginate to make room for new cells. Next, epithelial cells coordinate with underlying neuro-mesenchymal cells, and both tissues differentiate into the cells that make enamel, dentin, pulp, cementum, the PDL and alveolar bone. By 8 weeks (before the palate has fused), there are 10 buds in each dental lamina (the regions fated to become the maxillary and mandibular alveolar ridges). The tooth buds of permanent teeth begin forming shortly after the primary buds. Both primary and permanent buds develop into crowns and become surrounded by the maxilla and mandible, once they ossify.
Enamel is produced by cells derived from the ectoderm, whereas dentin, cementum, pulp, PDL and alveolar bone are derived from neuro-mesenchyme. We did not say mesoderm (one of the three embryonic tissue layers), but mesenchyme (the tissue type)—the difference is important. While the hard tissues enamel, dentin and cementum all share characteristics with bone tissue, enamel has bigger differences because of its lineage. Perhaps the biggest difference is that people cannot grow new enamel after teeth erupt. Enamel can passively re-mineralize under the right conditions, but this does not involve the activity of human cells or enzymes (which are a lot more efficient at producing enamel). The enamel-producing cells are lost during tooth eruption. Dentin, pulp, cementum and periodontal ligaments contain cells throughout the life of a tooth, and have a much higher capacity to undergo remodeling throughout life and regenerate following trauma.
The mesenchyme is special and worth attention. Most mesenchyme is derived from mesoderm, but tooth connective tissues are derived from neural crest cells that join mesoderm. This leads to some unexpected similarities in the behavior of tooth and brain cells. This makes less sense to people who haven’t learned embryology.
Lastly, enamel and dentin-producing cells are not induced to form separately. They induce one another in a reciprocal fashion. After this team-effort, the cells move apart from one another and do their own thing. Taken together, this reduces the chances that enamel or dentin could be produced in the wrong place or wrong time. They require a very special set of conditions that occurs only briefly during early embryonic development. Unfortunately, this also makes it harder for medical professionals to re-create these conditions later in life if more enamel or dentin would be beneficial.
Tooth formation

Tooth formation, or odontogenesis, starts with primary teeth beginning to form around weeks 6-7, and permanent teeth around weeks 9-10. At week 6, the oral cavity is lined by ectoderm-derived epithelium, and deep to that is mesenchyme. For the skin and most of the oral cavity, the layer of mesenchyme is derived from mesoderm. Teeth, however, grow from ridges of ectoderm from the first pharyngeal arch (the mandibular arch) and the maxillary process, along the occlusal border of what becomes the mandible and maxilla. The mesenchyme layer here contains neural crest cells that migrated from the neural tube. Hence, we say the connective tissues of the teeth are derived from neuro-mesenchyme (or ecto-mesenchyme). Remember, because neural crest cells came from the neural tube, and the neural tube invaginates from the ectoderm, these cells are ectodermal by lineage. They go through an ectodermal-mesenchymal transition and resemble mesenchyme derived from mesoderm under the microscope.
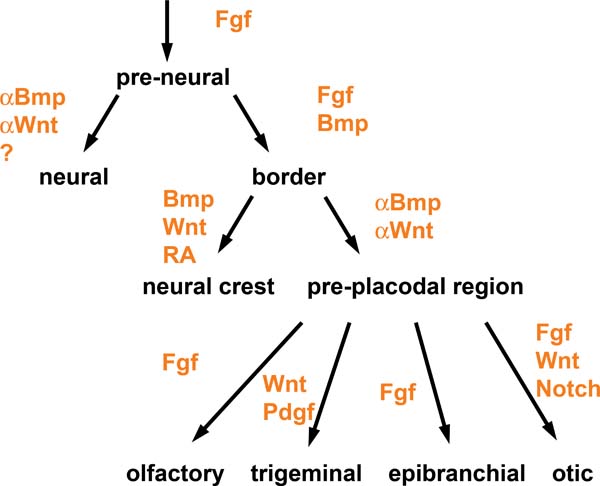
Induction
The first step is poorly understood. Neuro-mesenchymal cells coordinate with one another through planar cell polarity signals to ensure the correct number of tooth buds form, and at the correct distance apart from one another. These neuro-mesenchymal cells secrete a number of morphogens forming complex gradients, and overlying ectoderm respond to these morphogen gradients.

Two important morphogens in tooth development are members of the Fibroblast Growth Factor and the Bone Morphogenetic Protein families of signaling molecules. As you might have guessed from their names, these chemicals aren’t specific to tooth formation, they induce different cells to do different things at different times or locations. At this time and location, they induce ectoderm to differentiate into tooth buds instead of oral mucosa. Other morphogens inhibit placode formation, ensuring proper spacing exists between one placode and the next. One of the first visible responses in the ectoderm is proliferation– certain regions begin to grow thicker, and can at this time be called tooth placodes. Not every book (or exam) includes placode as its own stage, be aware. The placodes may be called the dental lamina or may be listed as a part of the dental lamina—fate mapping experiments can’t easily be done on human embryos to accurately distinguish between these two technicalities. The important concept is most of the oral mucosa described in chapter 3 is derived from ectoderm and mesoderm, while the periodontium—plus Junctional Epithelium—is derived from ectodermal and neuro-mesenchymal cells of a tooth bud.
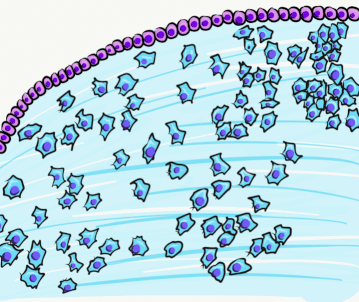
Bud stage
Proliferation of regions of ectoderm allows us to see the next stage of tooth development under the microscope, the bud stage. The name bud comes from the fact that tooth buds look like leaf buds on a plant. In the spring, you can see where leaves are fated to form on deciduous plants by the location of buds. Plant buds are not leaves yet, just bumps. Tooth buds begin to appear around week 6, and ultimately 10 tooth buds form on the maxillary processes and mandibular arch. Neuro-mesenchymal cells in the area also begin to proliferate. This makes the mesenchyme in a tooth bud appear different from the mesenchyme in regions that forms the sub-mucosa, those regions have more of the mucous ground substance and fewer cells.
Cap stage
Around week 10, the ectoderm continues to proliferate and it bumps into the cluster of neuro-mesenchymal stem cells below. This cluster of neuro-mesenchymal stem cells is called the dental papilla. The dental papilla forces the ectoderm of the tooth bud to grow around it, causing the ectoderm of the bud to resemble a cap (a hat), hence the name cap stage. At this time, the dental lamina has two prominent parts—an inner and an outer layer. The epithelial cells near the dental papilla are referred to as the enamel organ, because some of them differentiate into cells that produce enamel. The neuro-mesenchyme of the dental papilla forms dentin and pulp. The neuro-mesenchymal cells on the outside of the bell are called the dental sac (or dental follicle), which forms cementum, periodontal ligament and alveolar bone. All three together—the enamel organ, dental papilla and dental sac—are collectively called tooth germ.
At this time, the process repeats (or recapitulates). Cells of the dental lamina on the lingual side of the cap are induced to form another placode, and the succedaneous (permanent) teeth bud off the developing primary tooth germ. Imagine taking Fig 8.5, rotate it 90o, superimpose that onto the cap stage, a you have succedaneous tooth bud formation. Exceptions to this include the 2nd and 3rd molars, which do not succeed primary teeth. Their tooth buds develop from ectoderm similar to the primary dentition. The tooth germ of succedaneous teeth can move as they develop—tooth eruption does not always occur from the original lingual side, for instance the maxillary incisors generally erupt from the facial direction.
Bell stage

By the 11th-12th week, ectoderm and neuro-mesenchyme continues to proliferate. As the cap becomes larger, it resembles more of a bell-shape (bells on churches are usually larger than hats, may help you remember which stage comes before the other), hence the name bell stage. At this time, the enamel organ is two layers of cells named the inner enamel epithelium (IEE) and the outer enamel epithelium (OEE). The inner enamel epithelium is next to the dental papilla, with a basement membrane physically separating the two cell types. The IEE differentiates into ameloblasts and produce enamel, while the OEE is involved in tooth eruption and forms the junctional epithelium that bridges between the tooth surface and the oral mucosa.

Two more distinct regions of ectoderm develop at this time. The stratum intermedium is on the opposite side of the IEE from the dental papilla. These cells work with the IEE to form enamel. Past the stratum intermedium are ectodermal cells called the stellate reticulum, named for their star-shaped rather than cuboidal appearance. These cells help induce the IEE to differentiate and form enamel. These cells are found in the superficial but not the deeper regions of the growing tooth germ, this accounts for the production of enamel only in the crown and not the roots of teeth.
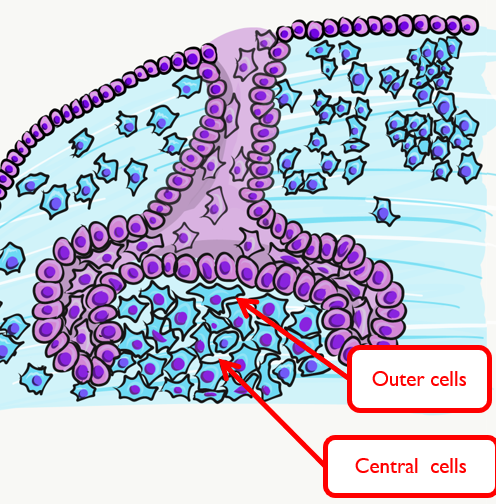
The dental papilla may be subdivided at this time into an outer region and a central region. The outer cells differentiate into odontoblasts, while the central cells form many cell types found in pulp. It is worth noting that cells are not ameloblasts or odontoblasts at this time. These cells are epithelial and neuro-mesenchymal stem cells, but based on their relative location, we know what they are fated to become in the near future, assuming they receive the correct morphogens.

A brief overview of the formation of ameloblasts and odontoblasts
During the bell stage, interactions between the IEE, stellate reticulum and outer cells of the dental papilla induce the differentiation of ameloblasts and odontoblasts. First, the basement membrane that physically separated the IEE from the neuro-mesenchymal cells of the dental papillae disintegrates. The IEE, now in contact with the neuro -mesenchymal cells and stellate reticulum, is induced to differentiate into pre-ameloblasts. The prefix pre- indicates that differentiation is not considered complete at this time, but the IEE begins to look different: the cells elongate, changing from simple cuboidal epithelium to a simple columnar epithelium. These cells produce morphogens that induce neighboring neuro-mesenchymal cells to differentiate into odontoblasts (the cells that make dentin). Newly formed odontoblasts begin producing a squishy immature form of dentin that mineralizes later. This pre-dentin, along with signals from the stellate reticulum, triggers the differentiation of pre-ameloblasts into ameloblasts (the cells that make enamel).

In developmental biology, this dual effort is known as reciprocal induction, which is a common pattern. This is significant because it makes it hard to re-create one type of cell later in life (such as an ameloblast) without also re-creating s second cell type (such as an odontoblast) as well as re-creating the conditions they were in during reciprocal induction (such as creating pre-ameloblasts and stellate reticulum).
Dentinogenesis, the formation of dentin, therefore begins shortly before amelogenesis, the formation of enamel. This results in dentin being thicker than enamel. As odontoblasts secrete pre-dentin, their cell bodies are pushed deeper, but they leave a log tube-like extension called the odontoblastic process within the pre-dentin. By the time a tooth is done growing, the odontoblastic processes extend through nearly the entire layer of mature dentin. Ameloblasts, on the other hand, do not leave behind an extension. There is a little bump on each Ameloblast facing the enamel, and this bump is called Tome’s process. There is important clinical significance related to odontoblastic processes coming up in subsequent chapters. Tome’s process, on the other hand, is a name of a bump histologists use to identify ameloblasts during embryonic development.
Crown stage
After the bell stage is the late bell stage, or the crown stage. During this time the crown of the teeth form by the secretion of molecules by ameloblasts and odontoblasts. No new cells of these types form, but teeth continue to grow by the addition of ECM. Because the matrix mineralizes and hardens, these tissues must be added appositionally. This is somewhat similar to the growth of bone tissue, only the enamel and dentin-producing cells do not get trapped between layers of hard tissue, they are pushed in an apical or basal direction. The crown portion of each primary tooth is finished growing sometime within the first year or two of life. The timeline for the succedaneous teeth is more variable (a timetable may be found on Wikipedia).
Root Development

The cervical loop
We jump years in time to watch the formation of the roots, after the crown is completed. The leading edge of the IEE and OEE are not separated by other cell types, together they are called the cervical loop. As the cervical loop continues to grow around the dental papillae and extend deeper, it is called Hertwig’s Epithelial Root Sheath (HERS). The pattern of HERS growth determines the shape of the root(s). HERS does not grow evenly around the dental papillae of larger teeth. Instead, HERS grows faster in some regions as it extends over the dental papilla, perhaps the way chocolate fudge drips over a ball of ice-cream unevenly. On larger teeth, this produces multiple roots.
Cementum formation
![[add-text output image]](https://med.libretexts.org/@api/deki/files/22976/add-text-output-image.gif?revision=1)
HERS contains IEE and OEE, no stellate reticulum, which leads to key differences between the crown and roots of teeth. The IEE induce the formation of odontoblasts in the roots just as it did in the crown, but when odontoblasts form pre-dentin, the lack of a signal from stellate reticulum means the differentiation of ameloblasts does not occur. Hence, roots do not contain enamel. Instead, most of the IEE and OEE cells undergo apoptosis. A few are left behind, which get the name the Epithelial Rests of Malassez (ERM). These leftover cells may have functions in the regeneration of damaged root tissues, although this is not currently well understood.
After the majority of the epithelial cells are removed, neuro-mesenchymal stem cells of the dental sac contact pre-dentin, and this induces them to differentiate into cementoblasts. These cells cover the root dentin in a relatively thin layer of cementum. Some regions of cementum have cementoblasts trapped within the ECM. This is cellular cementum, and the cells are called cementocytes once trapped within cementum. Other regions of cementum are acellular cementum. Formation of root dentin and cementum are covered in more detail in the next two chapters.
Tooth eruption

We now jump forward in time yet again. Even though process of tooth eruption happens long after embryological development, it should be considered a developmental process. Because this process involves cells of the IEE and OEE, we are discussing it here, rather than splitting up processes chronologically.
After the roots develop, neuro-mesenchymal stem cells of the dental sac that did not come into contact with dentin differentiate into fibroblasts and osteoblasts. These form the PDL and alveolar bone. Therefore, development of the PDL occurs during tooth eruption, much later than the formation of dentin and enamel.
First, the force or forces that cause teeth to erupt are not agreed upon. It is not even clear if it is a pushing or a pulling force, or a combination of the two. Whatever the cause or causes of tooth eruption are, movement of the crowns into the oral cavity is known as active eruption. One might imagine that as a root grows deeper and bumps into the edge of a bony socket, further lengthening of the root would force the crown upwards. This is similar to jumping: with your legs bent and feet on an immovable floor, rapid extension of your legs pushes you upwards. This is known as the root formation theory. To say this causes tooth eruption, it would have to be both necessary and sufficient (a phrase commonly used in biological research). Because sometimes root-less teeth erupt, this suggests root growth is not necessary for eruption.
We know that bone remodeling plays a role, which involves coordinated activity between osteoblasts and osteoclasts. We have already covered some of the evidence for this when we discussed how permanent teeth fail to erupt in people with cleidocranial dysostosis. A faulty matrix metalloproteinase enzyme leads to the inability to degrade ECM proteins, leaving permanent teeth trapped within their bony crypt. This suggests bone remodeling is necessary, but not necessarily sufficient. For the primary teeth, when growing roots encounter mineralized bones tissue, this puts hydrostatic pressure on the cells of the dental organ. In response, the stellate reticulum secretes morphogens that induce bone resorption, including RANKL, a morphogen that induces osteoclast differentiation, and Parathyroid Hormone, a hormone that activates osteoclast activity. The stellate reticulum, found at the surface of the developing crown enamel, therefor secretes a signal that clears a path for the tooth to erupt. Furthermore, as the roots grow, they put pressure on bone tissue in the alveolar sockets. Pressure on bone tissue triggers release of bone-simulating morphogens (BMPs) which leads to the deposition of bone tissue, pushing teeth outwards. Bite forces may also contribute to BMP secretion.
Further evidence that pressure is necessary comes from observation that teeth may fail to erupt in the absence of healthy periodontal ligament. In fact, pressure created by fibroblasts of the PDL may trigger the stellate reticulum to secrete morphogens that trigger osteoclast differentiation as well as bone tissue to release osteoblast-stimulating morphogens. Fibroblasts, after all, not only secrete collagen but can remove it as well, thus shortening of collagen fibers would put pressure on both the tooth root and alveolar socket. Polarization of PDL fibroblasts is observed before tooth eruption, suggesting that remodeling of PDL collagen fibers inserted in cementum may actively pull the tooth outwards.

Changes in the gingiva also occur for tooth eruption to proceed. Remodeling of oral mucosa to allow teeth to erupt is known as passive eruption. Old tissue is removed to clear a path for a tooth to erupt, but do not think of this like digging a tunnel for cars to pass through. The oral mucosa must be connected to the tooth as it erupts, otherwise oral bacteria could enter the sub-mucosa and trigger an infection or inflammatory response. Before eruption begins, the crown of the tooth contains 2 layers of epithelial cells on the surface of the enamel. This includes the ameloblasts and OEE. Collectively, these two layers of epithelial cells are called the Reduced Enamel Epithelium (REE). Like the invagination and separation of ectodermal cells during the formation of the neural tube, the REE is separated from the epithelial cells of the oral mucosa. During tooth eruption, the REE re-joins the oral epithelium, forming desmosomes and other cell-cell junctions with oral epithelial cells, but maintaining contact with enamel. Many REE cells undergo apoptosis, but those that remain become the junctional epithelium—a very special epithelium that maintains hemi-desmosome contacts on both sides of the tissue: apical (enamel) and basolateral (sub-mucosa).
Some of the REE may not fuse with the oral epithelium, and remain partially stuck to the surface of a newly erupted tooth. This is known as the primary enamel cuticle, or the more old-fashioned name Nasmyth’s membrane. It can easily be removed by gentle brushing or mastication.
Because the REE is lost with tooth eruption, no new enamel can be produced by human cells afterwards (amelogenesis is faster and more organized than passive re-mineralization because it involves a protein scaffold which assists in crystal formation). Furthermore, the lack of REE means that gingiva cannot form junctional epithelium with dental implants. Understanding how junctional epithelium forms in the first place may improve our ability to trick oral mucosa cells into becoming more like junctional epithelium.
Clinical considerations
Initiation stage complications
Aberrations in the initiation of tooth germ leads to the formation of too few or too many teeth.

Anodontia
Failure in the initiation stage results in missing teeth, or anodontia. This may include inadequate release of morphogens by neuro-mesenchymal stem cells, mutations in the morphogen receptor proteins, or teratogens that interfere with the binding of morphogens to their receptors and/or activation of second messenger systems. Partial anodontia (also called hypodontia), where one or a few teeth are missing, is most common. The most commonly missing teeth are: permanent maxillary lateral incisors, 3rd molars, and mandibular 2nd pre-molars.
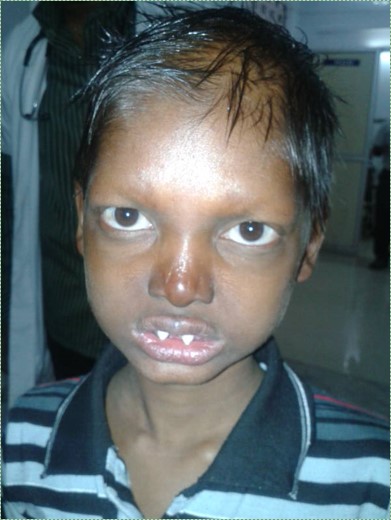
Ectodermal dysplasia (part 2 of 2)
Ectodermal dysplasia is covered in chapter 6 where we discuss the induction of neural crest cells. When signals from neural crest cells are reduced, regions of ectoderm that are supposed to grow faster than others do not get the boost in growth they need, including hair follicles, finger and toenails, tooth buds, sweat glands and salivary glands. This may cause some or all of these structures to not develop at all, or to develop in reduced number or size.
For those who cannot afford and/or are too young for dental implants, complete or partial anodontia may be visible, as well as microdontia (Fig. 8.16). Children do not receive dental implants because implants do not grow in size to match a growing jawline. Multiple sets of dentures are an option (again, for those who can afford the treatment). Now is a good time to double-check you can distinguish between hypodontia and microdontia. If you haven’t had Latin or a good medical terminology class, remember a hypo-dermic needle goes lower than the dermis, while micro-biology is the study of really small stuff.
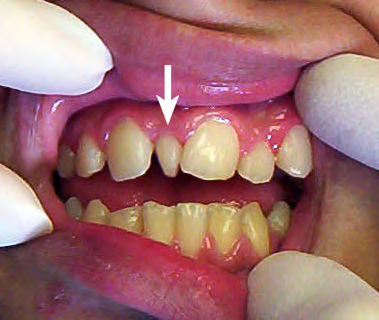
Hyperdontia
Hyperdontia, of the formation of extra (supernumerary) teeth, occurs when initiation of tooth germ occurs where it shouldn’t. This is often a genetic condition. The most common extra teeth are between the central incisors (mesiodens), distal to the maxillary 3rd molar (4th molar or distomolar) and the premolar region of either dental arch (perimolar).
Bud and cap stage complications
Aberrations in the bud stage of tooth development may lead to teeth that are too large (macrodontia) or too small (microdontia). This may affect all the teeth, or a single tooth.

Gemination
Gemination (twinning, as in the constellation Gemini) occurs when a single tooth germ is partially divided in two. This may occur if the growing tooth germ bumps into a small dense region in the dental arch. Under healthy conditions, teeth grow within loose mesenchyme tissue, the mesenchyme later differentiates into osseous tissue and calcifies. A geminated tooth, coming from a single tooth germ, is larger than average but has a single pulp cavity, hence this patient has an instance of macrodontia (but not hypodontia).
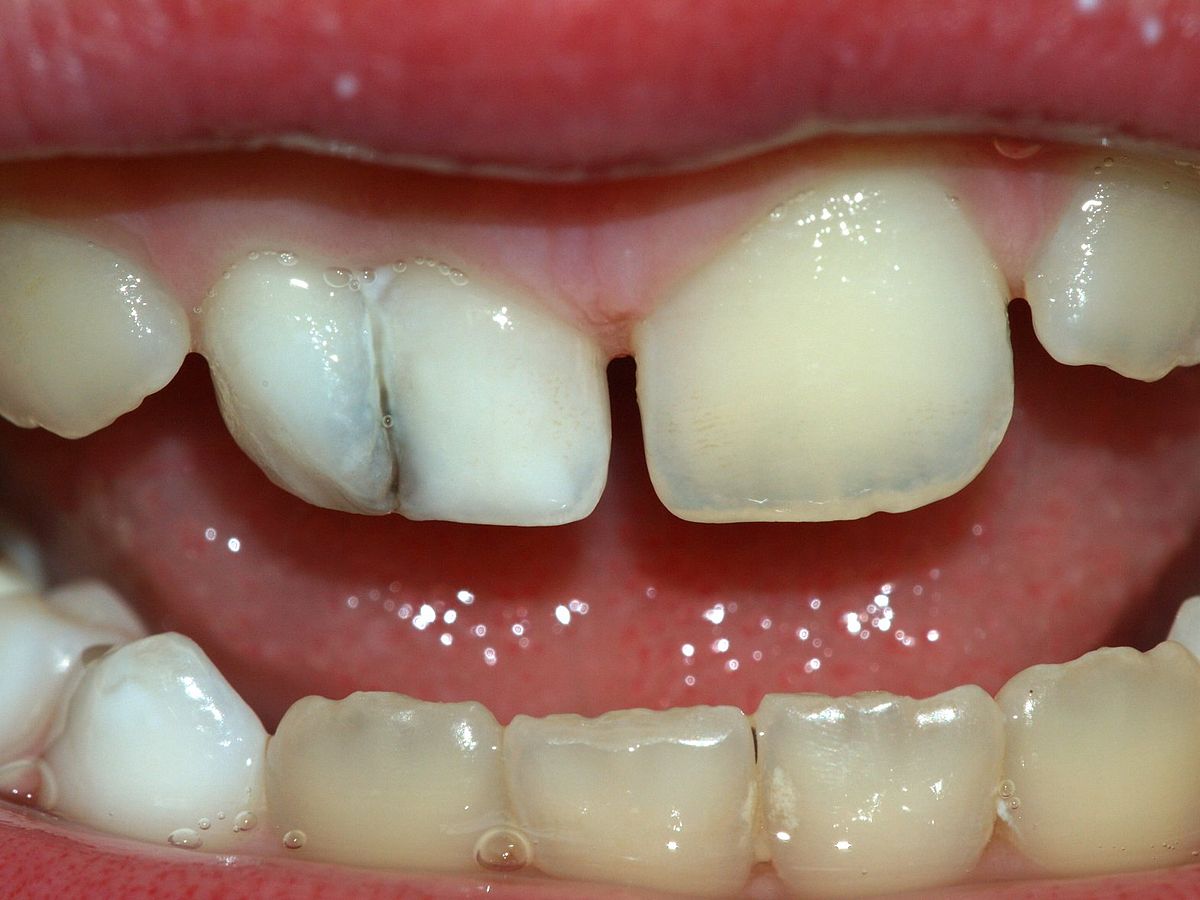
Fusion
Toothfusion (joining) of two tooth germs into a single germ can also cause a larger-than-average tooth to develop. In this case, macrodontia is accompanied by hypodontia (one fewer tooth). The fused tooth has two separate pulp cavities. This occurs when initiation of two tooth germs occurs too closely to one another, or when external pressure forces two tooth germs closer together. Tooth germs can fuse during early stages of tooth development because the tooth germ is soft epithelial and mesenchymal tissues. Getting too close together during later stages, when enamel and dentin are calcified, leads to plain-old tooth crowding (malocclusion).
It is worth a mention that the fusion of teeth involves two separate buds growing into what appears to be one large structure when their secretions meet. Syndactyly, the fusion of toes or fingers, is not really a fusion but a lack of separation due to diminished or absent apoptosis signals.

Dens in dente
Dens in dente (tooth within a tooth, or dens invaginitus) occurs when a small region of the enamel organ grows too fast and invaginates into the dental papilla during the cap stage. This sub-region of the enamel organ continues to develop, creating a miniature tooth within the main tooth. This can complicate a root canal surgery, but otherwise only makes for interesting radiographs.

Dilaceration
Dilaceration, or a bend in the shape of a tooth, occurs when there is an impediment to the growth of HERS. A delay in tooth eruption can cause dilaceration. The older part of the tooth (the crown), which has calcified, will have grown at a different angle from the newer, softer root(s) of the tooth if the tooth bumps into calcifying osseous tissue and is forced to change its direction of growth. Alternatively, trauma to a deciduous tooth can be transduced to the deeper succedaneous tooth, causing dilaceration of the permanent tooth (Fig 8.21).
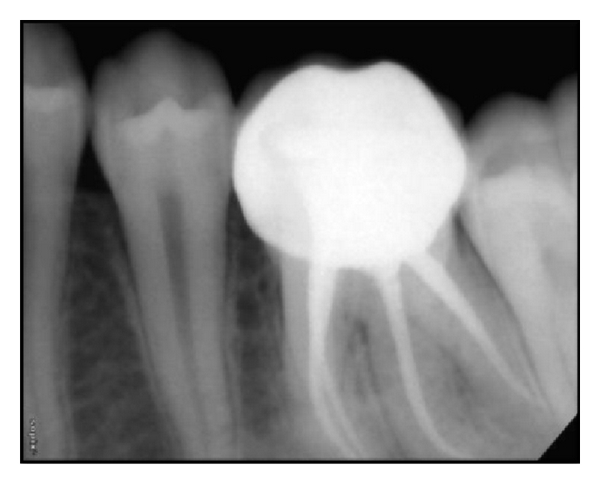
Supernumerary roots
Teeth may develop extra, or supernumerary roots. As with dens in dente, this may complicate root canal surgery, but otherwise has limited clinical significance. When the shape of the tooth is altered but no pathology results, clinicians and textbook authors may note there is an alteration to the morphology of the tooth.
Eruption complications

Teething
During tooth eruption, several tissues undergo significant remodeling, including the REE, oral epithelium and bone tissue. This involves removal of ECM by the secretion of digestive enzymes, and removal of cells by apoptosis. However, enzymes aren’t smart enough to only remove ECM, they tend to kill cells as well. For instance, when the enamel organ releases proteinases to clear a path for the erupting tooth, this triggers inflammation as cell membrane proteins are destroyed along with ECM, leading to cell death. Inflammation can cause discomfort, tenderness or edema in the affected area. Rubbing a baby’s gums can reduce pain signals from being sent by nociceptors (similar to rubbing your knee after you hit it on something). Alternately, cooling the area reduces inflammation. Placing a teething ring in the refrigerator (not freezer) can be used to induce cooling in the affected area. If those techniques do not provide adequate comfort, a children’s dose of over-the-counter anti-inflammatory medication may be administered.
It is not recommended to give babies the stronger over-the-counter pain-killing medication lidocaine. This medication is safe for use by adults to treat oral cavity pain, such as that caused by ulcers. However, it is significantly harder to measure a safe dose for a small baby than a large adult. Furthermore, because the nervous system of a newborn is still developing, there is always a risk that these medications will interfere with development, much the same way medications can act as teratogens during embryonic development. Developing the correct connections between axons and dendrites, whether in embryonic development or in childhood, involves multiple morphogens and electrical activity of neurons, as illustrated by the seminal work of neuroscientist Mu-Ming Poo. Lidocaine blocks electrical activity.
The use of homeopathic teething tablets or gels is not recommended. These products provide no measured benefit, and their contents are unregulated. Furthermore, in 2010 the FDA forced the recall of one such product (a rare event thanks to legal protections for the alternative and/or complementary medicine industry) because it contained belladonna (deadly nightshade). Teething necklaces or other jewelry are also not recommended because they provide no measured benefit but introduce a choking hazard.

Primary failure of eruption
Primary failure of eruption (PFE) is the partial or complete failure of a tooth to erupt (otherwise known as an impacted tooth) despite a healthy eruption pathway. While there are likely many causes of PFE, one major heritable cause is a mutation that disrupts Parathyroid Hormone signaling from the tooth germ to alveolar bone tissue. Parathyroid hormone activates osteoclasts and inhibits osteoblasts, therefore inhibiting parathyroid hormone signals leads to increased bone deposition. This leads to ankylosis (stiffening or immobilizing) of the tooth prior to eruption, making extracting difficult or impossible, especially as time progresses. Impacted teeth may cause inflammation and pain, or may not be detected until an x-ray is performed. The 3rd molars are the most commonly impacted teeth. Complications include crowding of neighboring teeth, infection, malocclusion, and– rarely but significantly– neoplasia, making extraction important.
Mechanical failure of eruption
A tooth may also become impacted after erupting partially because of ankylosis of the tooth, which is termed mechanical failure of eruption (MFE—which seems odd because it pairs a numerical name with a descriptive name). In contrast to PFE, there is a physical barrier to the eruption pathway. Absence of the PDL indicates fusion of tooth to alveolar bone and can indicate MFE. With MFE, more so than PFE, orthodontic or other therapies may allow the impacted tooth to erupt eliminating the need for extraction and replacement.
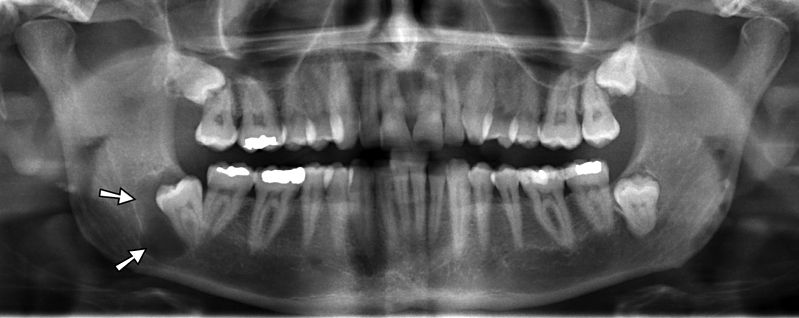
Dentigerous cyst
An impacted tooth can produce a cyst around the crown, called a dentigerous cyst. As the motionless tooth attempts to digest its way through oral epithelium, fluid accumulates between the crown and REE. The cyst may continue to grow in size, causing pain and trauma to the jaw bone (usually the mandible). Extraction of the impacted tooth will solve the issue, or orthodontic facilitation of tooth eruption may be an option as well. Rarely, the REE in a dentigerous cyst develops into a benign tumor known as an ameloblastoma. The main risk of this cancer is that similar to that of the cyst—it can cause pressure which may cause bones to break or to grow malformed. The reason this tumor is rare is that the REE contains differentiated (non-mitotic) cells. Because these epithelial cells are anchored to one another by desmosomes, this type of tumor rarely metastasizes.
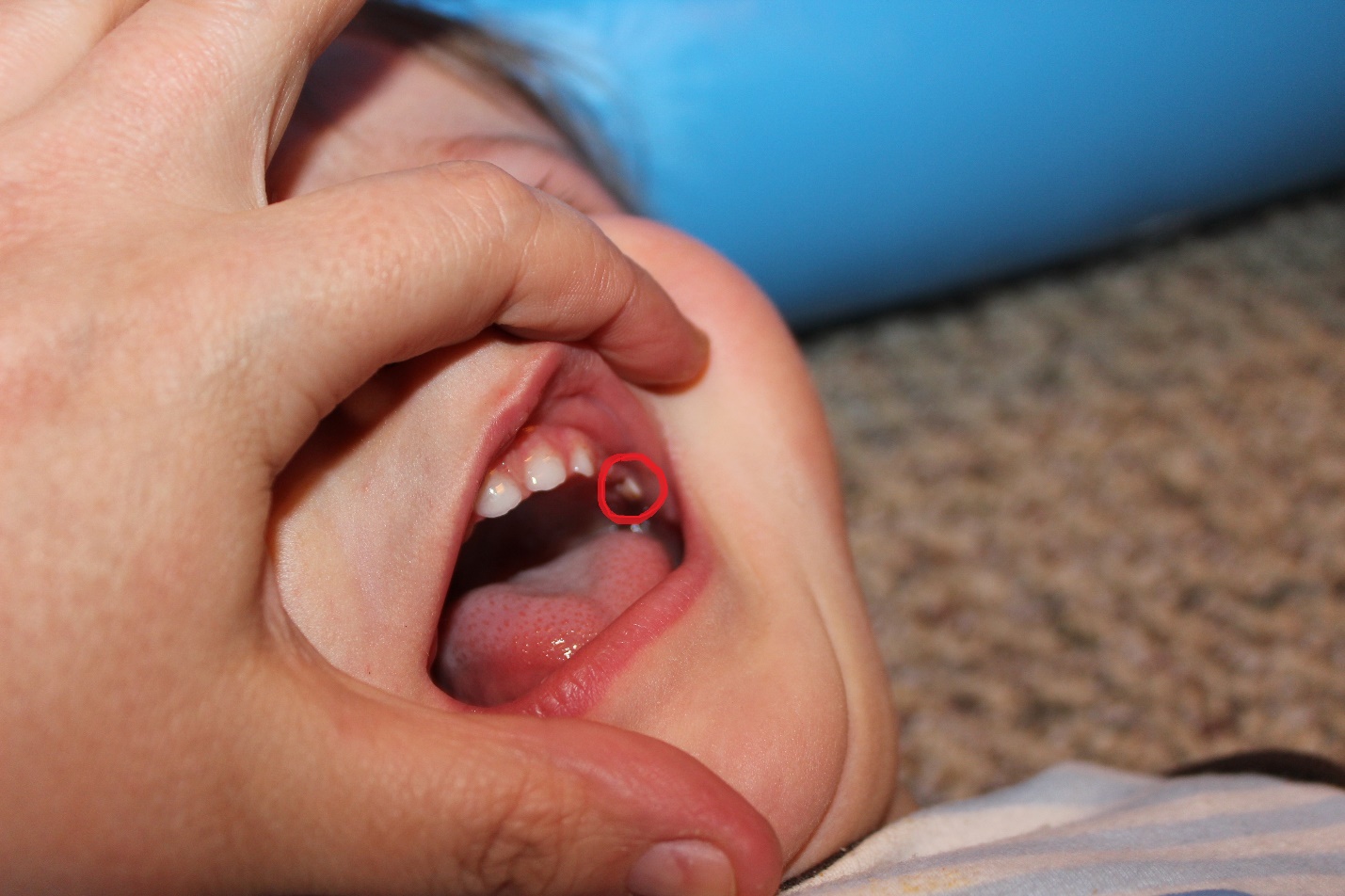
Bohn’s nodules
Bohn’s nodules (referred to as epithelial rests of Serres in older texts) are another gingival cyst similar to Epstein pearls. They are masses of keratin trapped in the gingiva, produced by the REE rather than oral epithelium. Sometimes remnants of minor salivary glands are also called Bohn’ nodules, despite a lack of keratin. Their presence along the alveolar ridge may cause parents to mistake them for erupting teeth. They more commonly occur along the maxillary ridge. Like Epstein pearls, these cysts are transient—they go away on their own, usually within 3 months, and produce few in any disturbances.
Lastly, if you are interested in the history of embryology and long-dead European men, we offer the following. Antoine Étienne Serres collaborated with the embryologist Johann Friedrich Meckel, who named the cartilage in the first pharyngeal arch that develops into the mandible (Meckel’s cartilage). Together, they attempted to unify embryology and the lineage of different species (late called evolution by heritable selection by Charles Darwin and the less-well-known Alfred Russel Wallace). Their theory was later developed by Ernst Haeckel into the famous phrase “ontogeny recapitulates phylogeny”, which we have quoted earlier.
Sadly, while Haeckel is celebrated for his work as an embryologist (including in this book), he also published racist pseudoscience which influenced the eugenics movements in the U.S. and Nazi Germany. This may seem like a distraction, but the idea of a biological definition of race is still popular in the U.S. and elsewhere, despite having been clearly disproved by the human genome project. Furthermore, the rate of untreated caries is triple for African Americans children compared to their Caucasian peers in the U.S. thanks to ongoing systemic racism. This disparity persists into adulthood, affecting the likelihood of receiving endodontic therapy and other dental procedures. This is not due to differences in genes (nature), it is due to inequality in dental care (nurture).
Chapter 7 * Chapter 9