13.4: Vision
- Page ID
- 63450
\( \newcommand{\vecs}[1]{\overset { \scriptstyle \rightharpoonup} {\mathbf{#1}} } \)
\( \newcommand{\vecd}[1]{\overset{-\!-\!\rightharpoonup}{\vphantom{a}\smash {#1}}} \)
\( \newcommand{\id}{\mathrm{id}}\) \( \newcommand{\Span}{\mathrm{span}}\)
( \newcommand{\kernel}{\mathrm{null}\,}\) \( \newcommand{\range}{\mathrm{range}\,}\)
\( \newcommand{\RealPart}{\mathrm{Re}}\) \( \newcommand{\ImaginaryPart}{\mathrm{Im}}\)
\( \newcommand{\Argument}{\mathrm{Arg}}\) \( \newcommand{\norm}[1]{\| #1 \|}\)
\( \newcommand{\inner}[2]{\langle #1, #2 \rangle}\)
\( \newcommand{\Span}{\mathrm{span}}\)
\( \newcommand{\id}{\mathrm{id}}\)
\( \newcommand{\Span}{\mathrm{span}}\)
\( \newcommand{\kernel}{\mathrm{null}\,}\)
\( \newcommand{\range}{\mathrm{range}\,}\)
\( \newcommand{\RealPart}{\mathrm{Re}}\)
\( \newcommand{\ImaginaryPart}{\mathrm{Im}}\)
\( \newcommand{\Argument}{\mathrm{Arg}}\)
\( \newcommand{\norm}[1]{\| #1 \|}\)
\( \newcommand{\inner}[2]{\langle #1, #2 \rangle}\)
\( \newcommand{\Span}{\mathrm{span}}\) \( \newcommand{\AA}{\unicode[.8,0]{x212B}}\)
\( \newcommand{\vectorA}[1]{\vec{#1}} % arrow\)
\( \newcommand{\vectorAt}[1]{\vec{\text{#1}}} % arrow\)
\( \newcommand{\vectorB}[1]{\overset { \scriptstyle \rightharpoonup} {\mathbf{#1}} } \)
\( \newcommand{\vectorC}[1]{\textbf{#1}} \)
\( \newcommand{\vectorD}[1]{\overrightarrow{#1}} \)
\( \newcommand{\vectorDt}[1]{\overrightarrow{\text{#1}}} \)
\( \newcommand{\vectE}[1]{\overset{-\!-\!\rightharpoonup}{\vphantom{a}\smash{\mathbf {#1}}}} \)
\( \newcommand{\vecs}[1]{\overset { \scriptstyle \rightharpoonup} {\mathbf{#1}} } \)
\( \newcommand{\vecd}[1]{\overset{-\!-\!\rightharpoonup}{\vphantom{a}\smash {#1}}} \)
\(\newcommand{\avec}{\mathbf a}\) \(\newcommand{\bvec}{\mathbf b}\) \(\newcommand{\cvec}{\mathbf c}\) \(\newcommand{\dvec}{\mathbf d}\) \(\newcommand{\dtil}{\widetilde{\mathbf d}}\) \(\newcommand{\evec}{\mathbf e}\) \(\newcommand{\fvec}{\mathbf f}\) \(\newcommand{\nvec}{\mathbf n}\) \(\newcommand{\pvec}{\mathbf p}\) \(\newcommand{\qvec}{\mathbf q}\) \(\newcommand{\svec}{\mathbf s}\) \(\newcommand{\tvec}{\mathbf t}\) \(\newcommand{\uvec}{\mathbf u}\) \(\newcommand{\vvec}{\mathbf v}\) \(\newcommand{\wvec}{\mathbf w}\) \(\newcommand{\xvec}{\mathbf x}\) \(\newcommand{\yvec}{\mathbf y}\) \(\newcommand{\zvec}{\mathbf z}\) \(\newcommand{\rvec}{\mathbf r}\) \(\newcommand{\mvec}{\mathbf m}\) \(\newcommand{\zerovec}{\mathbf 0}\) \(\newcommand{\onevec}{\mathbf 1}\) \(\newcommand{\real}{\mathbb R}\) \(\newcommand{\twovec}[2]{\left[\begin{array}{r}#1 \\ #2 \end{array}\right]}\) \(\newcommand{\ctwovec}[2]{\left[\begin{array}{c}#1 \\ #2 \end{array}\right]}\) \(\newcommand{\threevec}[3]{\left[\begin{array}{r}#1 \\ #2 \\ #3 \end{array}\right]}\) \(\newcommand{\cthreevec}[3]{\left[\begin{array}{c}#1 \\ #2 \\ #3 \end{array}\right]}\) \(\newcommand{\fourvec}[4]{\left[\begin{array}{r}#1 \\ #2 \\ #3 \\ #4 \end{array}\right]}\) \(\newcommand{\cfourvec}[4]{\left[\begin{array}{c}#1 \\ #2 \\ #3 \\ #4 \end{array}\right]}\) \(\newcommand{\fivevec}[5]{\left[\begin{array}{r}#1 \\ #2 \\ #3 \\ #4 \\ #5 \\ \end{array}\right]}\) \(\newcommand{\cfivevec}[5]{\left[\begin{array}{c}#1 \\ #2 \\ #3 \\ #4 \\ #5 \\ \end{array}\right]}\) \(\newcommand{\mattwo}[4]{\left[\begin{array}{rr}#1 \amp #2 \\ #3 \amp #4 \\ \end{array}\right]}\) \(\newcommand{\laspan}[1]{\text{Span}\{#1\}}\) \(\newcommand{\bcal}{\cal B}\) \(\newcommand{\ccal}{\cal C}\) \(\newcommand{\scal}{\cal S}\) \(\newcommand{\wcal}{\cal W}\) \(\newcommand{\ecal}{\cal E}\) \(\newcommand{\coords}[2]{\left\{#1\right\}_{#2}}\) \(\newcommand{\gray}[1]{\color{gray}{#1}}\) \(\newcommand{\lgray}[1]{\color{lightgray}{#1}}\) \(\newcommand{\rank}{\operatorname{rank}}\) \(\newcommand{\row}{\text{Row}}\) \(\newcommand{\col}{\text{Col}}\) \(\renewcommand{\row}{\text{Row}}\) \(\newcommand{\nul}{\text{Nul}}\) \(\newcommand{\var}{\text{Var}}\) \(\newcommand{\corr}{\text{corr}}\) \(\newcommand{\len}[1]{\left|#1\right|}\) \(\newcommand{\bbar}{\overline{\bvec}}\) \(\newcommand{\bhat}{\widehat{\bvec}}\) \(\newcommand{\bperp}{\bvec^\perp}\) \(\newcommand{\xhat}{\widehat{\xvec}}\) \(\newcommand{\vhat}{\widehat{\vvec}}\) \(\newcommand{\uhat}{\widehat{\uvec}}\) \(\newcommand{\what}{\widehat{\wvec}}\) \(\newcommand{\Sighat}{\widehat{\Sigma}}\) \(\newcommand{\lt}{<}\) \(\newcommand{\gt}{>}\) \(\newcommand{\amp}{&}\) \(\definecolor{fillinmathshade}{gray}{0.9}\)- Explain the role of the accessory structures of the eye.
- Describe the gross and microscopic structures responsible for the special sense of vision
- Trace the pathway of visual information from the photoreceptors to the brain
- Explain topographical representations of visual information
Vision
Vision is the special sense of sight that is based on the detection and transduction of light stimuli from the eyes to the brain. The eye contains the receptors for light (photoreceptors) that detect light, color (wavelength), and movement.
Eye Structure
The eye itself is a hollow spherical organ that measures approximately 2.5 centimeters across in a human. Within the orbit it is surrounded by adipose tissue, which serves as the "packing peanuts" that keep the eye in place and cushioned so that it does not shift position or contact the surrounding bone. The wall of the eye is composed of three layers called tunics (Figure \(\PageIndex{1}\) and Figure \(\PageIndex{2}\)).
Tunics
The outermost layer is the fibrous tunic, which includes the white sclera posteriorly and clear cornea anteriorly. The sclera accounts for five sixths of the surface of the eye, most of which is not visible, though humans are unique compared with many other species in having so much of the “white of the eye” visible. Think about your dog or cat - you do not see the whites of their eyes! The tough sclera (skleros = hard) is composed of dense irregular connective tissue. Functionally it give shapes to the eye and serves at the attachment point for the extraocular muscles (see chapter 9 for review). The transparent cornea covers the anterior surface of the eye. Its convex shape allows for the bending of light as it enters the eye, so any distortions of its shape will result in the inability to focus what you are looking at.

The middle layer of the eye is the vascular tunic, which is composed of the choroid, ciliary body, and iris. The choroid is a layer of highly vascularized connective tissue that provides a blood supply to the eyeball. This layer is also highly pigmented, with numerous melanocytes, in order to absorb any extraneous light and prevent it from reflecting within the spherical eye. The choroid is posterior to the ciliary body, a muscular structure that is attached to the lens by suspensory ligaments. Contraction and relaxation of the ciliary muscle alters the thickness and curve of the lend, allowing it to adjust the focal point of light on the back of the eye. Anterior to the the ciliary body, and visible externally, is the iris—the colored part of the eye. The color of the iris is dependent upon multiple factors related to melanin production, but the color runs from lower melanin (blue) to high melanin (dark brown). What appears as a black circle in the center of the iris is the pupil, which is really a hole in the center the through which light passes. The function of the iris is to adjust the amount of light entering the eye, so the smooth muscles within it will contract/relax in order to change the pupil diameter.
The innermost layer of the eye is the neural tunic, the retina, that contains the nervous tissue responsible for absorbing light and converting it into a neural signal. The retina consists of two layers: a pigmented layer and a neural layer. The pigmented epithelium is a single layer of pigmented cuboidal cells containing melanin that absorb light after it passes through the retina. These cells also provide vital metabolic support for the photoreceptors. The neural layer consists of three main sublayers: the photoreceptor layer, a bipolar cell layer, and the ganglion cell layer. The structure of the retina will be discussed more below.

Cavities and Chambers
The interior of the eye is divided into two cavities (Figure \(\PageIndex{3}\)) that are filled with circulating fluids: the anterior cavity and the posterior cavity. The anterior cavity is the space between the cornea and lens that is further divided into anterior and posterior chambers by the iris. These chambers are filled with a watery fluid called the aqueous humor. The posterior cavity is the space between the lens and the the retina that is filled with a thicker fluid called the vitreous humor. The vitreous humor has a similar consistency to the white part of an egg.

Retinal Structure
The retina is composed of several layers and contains specialized cells for the initial processing of visual stimuli. The photoreceptors change their membrane electrical properties when stimulated by light energy. Light falling on the retina causes chemical changes to pigment molecules in the photoreceptors. There are two types of photoreceptors called rods and cones (Figures \(\PageIndex{4}\) and \(\PageIndex{5}\). Cones each contain one of three photopigments that are specialized to absorb three different color ranges of the light spectrum: red, green, and blue. Rods can absorb light from across the color spectrum and they are able to function in low light conditions, for example in a darkened room. Cones need more light to function than rods, which is why you cannot see colors in a dimly lit environment. The absorption of light alters the amount of neurotransmitter the photoreceptor cells release onto bipolar cells (see Figure \(\PageIndex{16}\). Each bipolar cell receives signals from multiple photoreceptors. Multiple bipolar cells then pass their signal to a ganglion cell.


The axons of ganglion cells, which form the deepest layer of the retina, located adjacent to the posterior cavity, collect at the optic disc and leave the eye as the optic nerve. Because these axons exit through the retina, there are no photoreceptors at the location where the optic nerve begins (Figure \(\PageIndex{6}\)). This creates a “blind spot” in the retina, and a corresponding blind spot in our visual field.


Note that the photoreceptors in the retina (rods and cones) are located behind the axons, ganglion cells, bipolar cells, and retinal blood vessels. A significant amount of light is absorbed by these structures before the light reaches the photoreceptor cells. However, at the exact center of the retina is a small region known as the macula lutea at which center there is a depression called the fovea (Figure \(\PageIndex{7}\)). In these regions, the retina lacks the supporting cells and blood vessels, and only contains cones. Therefore, visual acuity, or the sharpness of vision, is greatest at the fovea. As one moves in either direction from this central point of the retina, visual acuity drops significantly. In addition, each photoreceptor cell of the fovea is connected to a single ganglion cell. Therefore, this ganglion cell does not have to integrate inputs from multiple photoreceptors, which reduces the accuracy of visual transduction. Toward the edges of the retina, several photoreceptors converge on retinal ganglion cells (through the bipolar cells) up to a ratio of 50 to 1. The difference in visual acuity between the fovea and peripheral retina is easily evidenced by looking directly at a word in the middle of this paragraph. The visual stimulus in the middle of the field of view falls on the fovea and is in the sharpest focus. Without moving your eyes off that word, notice that words at the beginning or end of the paragraph are not in focus. The images in your peripheral vision are focused by the peripheral retina, and have vague, blurry edges and words that are not as clearly identified. As a result, a large part of the neural function of the eyes is concerned with moving the eyes and head so that important visual stimuli are centered on the fovea.


Retina: Macular degeneration
Macular degeneration is a common age-related eye condition that affects central vision due to a progressive deterioration of the macula (Figure \(\PageIndex{8}\)). In early stages vision is not affected, but as the degeneration progresses central vision will become blurry or wavy. In late stages the central vision fails completely. People with macular degeneration aren’t completely blind as only the central vision is affected, peripheral vision (ability to see things off to the sides) is fine. Macular degeneration typically occurs in people over the age of 50, so it is considered age-related. The cause of this condition is unknown, but, since it runs in families, it can have a genetic component. Other risk factors include being overweight, smoking, having high blood pressure (hypertension), eating a diet high in saturated fats, and Caucasian ancestry.


Visual Pathways
The visual information of the optic nerve will be transmitted through the thalamus and sent to the primary visual cortex of the occipital lobe (Figure \(\PageIndex{9}\)). Prior to reaching the thalamus, some of the axons cross over to the opposite side at the optic chiasm. This occurs because each eye sees a part of both the right visual field (everything to the right of your nose) and of the left visual field (everything to the left of your nose). In order for the occipital lobes each to process all of the information from a single visual field, some of the information needs to head to the opposite side. After this crossing, the nerve fibers from a single field of view will travel in an optic tract and synapse on interneurons in the lateral geniculate nucleus of the thalamus. The thalamic axons will travel to the occipital lobe in a tract called the optic radiations.

Visual Pathway: Bitemporal hemianopia
Visual field deficits can be disturbing for a patient. Bitemporal hemianopia is a type of partial blindness where vision is missing in the lateral half of both the right and left visual fields (Figure \(\PageIndex{8}\)). One cause of this condition is growth of the pituitary gland that presses against the optic chiasm and interferes with signal transmission of the crossing fibers. The axons projecting to the same side of the brain are unaffected. Therefore, the patient loses the outermost areas of their field of vision and cannot see objects to their right and left yet they retain their central vision.


Cerebrum
The topographic relationship between the retina and the visual cortex is maintained throughout the visual pathway. The visual field is projected onto the two retinae, as described above, with sorting at the optic chiasm. The right peripheral visual field falls on the medial portion of the right retina and the lateral portion of the left retina. The right medial retina then projects across the midline through the optic chiasm. This results in the right visual field being processed in the left visual cortex. Likewise, the left visual field is processed in the right visual cortex. Though the chiasm is helping to sort right and left visual information, superior and inferior visual information is maintained topographically in the visual pathway. Light from the superior visual field falls on the inferior retina, and light from the inferior visual field falls on the superior retina. This topography is maintained such that the superior region of the visual cortex processes the inferior visual field and vice versa. Therefore, the visual field information is inverted and reversed as it enters the visual cortex—up is down, and left is right (Figure \(\PageIndex{11}\)). However, the cortex processes the visual information such that the final conscious perception of the visual field is correct. The topographic relationship is evident in that information from the foveal region of the retina is processed in the center of the primary visual cortex. Information from the peripheral regions of the retina are correspondingly processed toward the edges of the visual cortex. Similar to the exaggerations in the sensory homunculus of the somatosensory cortex, the foveal-processing area of the visual cortex is disproportionately larger than the areas processing peripheral vision.
In an experiment performed in the 1960's, subjects wore prism glasses so that the visual field was inverted before reaching the eye. On the first day of the experiment, subjects would duck when walking up to a table, thinking it was suspended from the ceiling. However, after a few days of acclimation, the subjects behaved as if everything were represented correctly. Therefore, the visual cortex is somewhat flexible in adapting to the information it receives from our eyes.
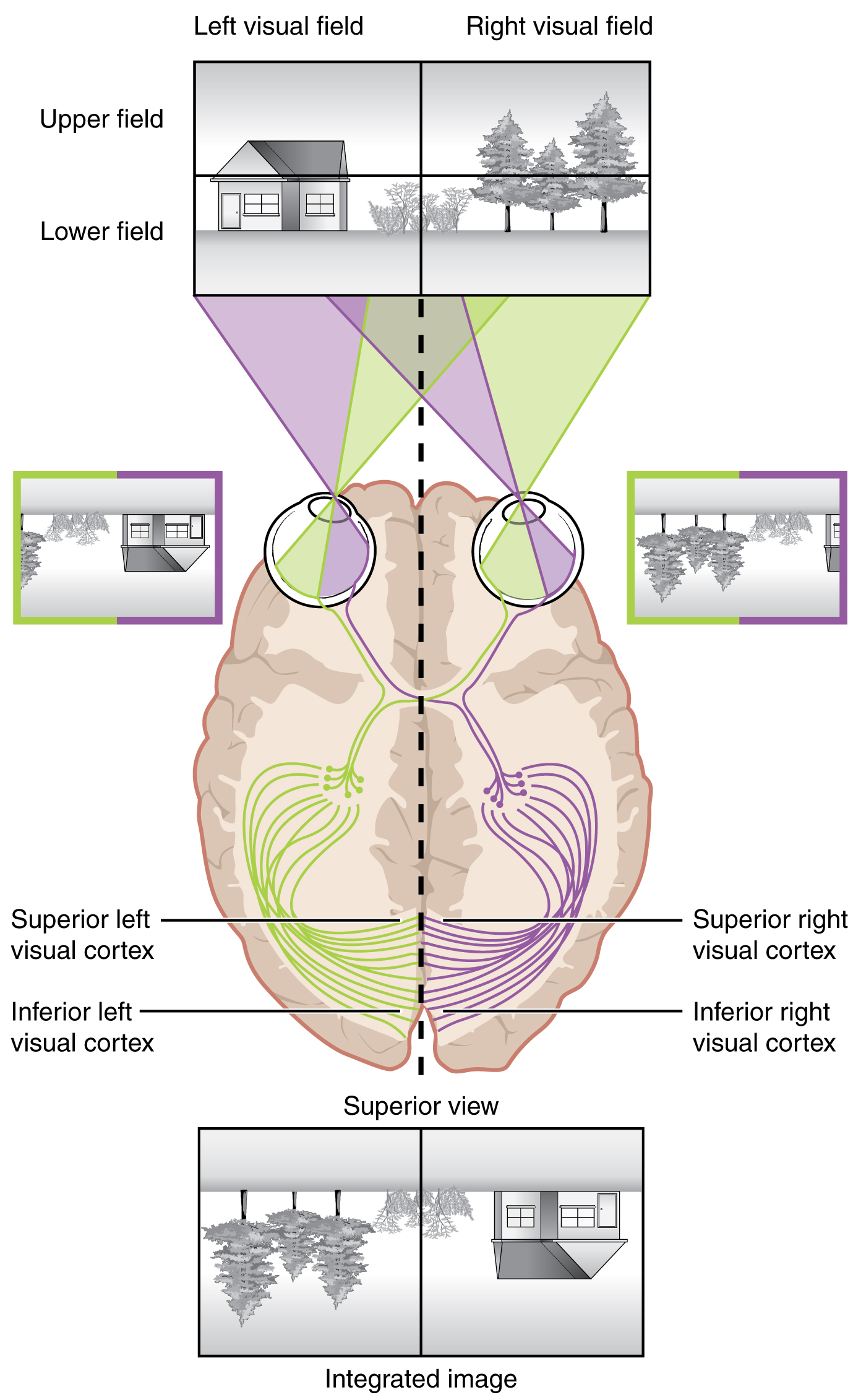
The cortex has been described as having specific regions that are responsible for processing specific information; there is the visual cortex, somatosensory cortex, gustatory cortex, etc. However, our experience of these senses is not divided. Instead, we experience what can be referred to as a seamless percept. Our perceptions of the various sensory modalities—though distinct in their content—are integrated by the brain so that we experience the world as a continuous whole.
In the cerebral cortex, sensory processing begins at the primary sensory cortex, then proceeds to an association area, and finally, into a multimodal integration area. For example, the visual pathway projects from the retinae through the thalamus to the primary visual cortex in the occipital lobe. This area is primarily in the medial wall within the longitudinal fissure. Here, visual stimuli begin to be recognized as basic shapes. Edges of objects are recognized and built into more complex shapes. Also, inputs from both eyes are compared to extract depth information. Because of the overlapping field of view between the two eyes, the brain can begin to estimate the distance of stimuli based on binocular depth cues.
Accessory Structures of the Eye
The accessory structures provide a superficial covering over the anterior surface of the eye. They serve to protect the eye from physical damage, prevent objects from entering the eye socket, and keep the exposed surface lubricated and moist (Figure \(\PageIndex{12}\)).
- The bony orbits surround the eyes, encasing them in a hard surrounding shell and anchoring the soft tissues of the eye in place.
- The eyelids, with lashes at their leading edges, help to protect the eye from abrasions by blocking and catching particles that may land on the surface of the eye. Each eyelid contains a thin covering of skin that, when closed, helps to distribute the lacrimal fluid (tears) to wash and lubricate the surface.
- The conjunctiva forms a continuous lining from the posterior side of the eyelid across the white portion of the eye. Made of stratified squamous epithelial tissue, the conjunctiva contains secretory cells, free nerve endings, and blood vessels.

Lacrimal Apparatus
The lacrimal apparatus associated with each eye is responsible for the production and drainage of lacrimal fluid (tears). The lacrimal fluid helps protect the eye by reducing friction of the eyelids, continuously cleaning the anterior surface of the eye, and preventing bacterial infection through the action of lysozyme. Tears are produced by the lacrimal gland, located within the superolateral depression of each orbit (Figure \(\PageIndex{13}\)). Tears produced by this gland are dispersed on the external surface of the eye by the movement of the eyelids and then flow towards the medial surface of the eye. Small openings drain the lacrimal fluid into channels that deliver it into the nasal cavity where it mixes with the mucus.

Extraocular Muscles
Movement of the eye within the orbit is accomplished by the contraction of six extraocular muscles that originate from the bones of the orbit and insert into the surface of the eyeball (Figure \(\PageIndex{14}\)). Four muscles run straight from a common tendinous ring in the posterior of the orbit and insert into the surface of the eye. The insertion side of each of these muscles is in its name: the superior rectus, the medial rectus, the inferior rectus, and the lateral rectus. Contraction of one of these muscles causes movement toward the corresponding side. For example, when the superior rectus contracts, the eye rotates superiorly causing you to look up. The remaining two extraocular muscles run at an angle and cause eye rotation either clockwise or counter clockwise. The superior oblique originates at the posterior orbit, near the origin of the four rectus muscles. However, the tendon of the superior oblique muscles threads through a pulley-like piece of cartilage known as the trochlea. The tendon inserts obliquely into the superior surface of the eye. The angle of the tendon through the trochlea means that contraction of the superior oblique abducts, depresses, and internally rotates (intorsion) the eye. The inferior oblique muscle originates from the floor of the orbit and inserts into the inferolateral surface of the eye. When it contracts, it abducts, elevates, and externally rotates (extorsion) the eye. Rotation of the eye by the two oblique muscles is necessary because the eye is not perfectly aligned on the sagittal plane. When the eye looks up or down, the eye must also rotate slightly to compensate for the superior rectus pulling at approximately a 20-degree angle, rather than straight up. The same is true for the inferior rectus, which is compensated by contraction of the inferior oblique. A seventh muscle in the orbit is the levator palpebrae superioris, which is responsible for elevating and retracting the upper eyelid, a movement that usually occurs in concert with elevation of the eye by the superior rectus.
The extraocular muscles are innervated by three cranial nerves:
- the lateral rectus, which causes abduction of the eye, is innervated by the abducens nerve (CN VI),
- the superior oblique is innervated by the trochlear nerve (CN IV), and
- the superior rectus, inferior rectus, inferior oblique, and medial rectus are innervated by the oculomotor nerve (CN III), as is the levator palpebrae superioris.

Figure \(\PageIndex{14}\): Extraocular Muscles. The extraocular muscles move the eye within the orbit. A lateral view of the eye shows the common tendinous ring, where the four rectus muscles originate from. The superior oblique muscle originate from the posterior orbit and its tendon threads through the trochlea to insert into the superior surface of the eye. The inferior oblique muscle originate from the inferior portion of the orbit and inserts into the inferolateral surface of the eye. An anterior view of the right eye shows the movements of the extraocular muscles. (Image credit: "Extraocular Muscles" by Jennifer Lange is licensed under CC BY-NC-SA 4.0, based on originals "Slagter - Drawing Sagittal section of the eye and muscles of eye - no labels" by Ron Slagter, license: CC BY-NC-SA and "Cenveo - Drawing Muscles that Control Eye Movement - English labels" by Cenveo, license: CC BY.)
Concept Review
Vision belongs to the special senses and its specialized organ is the eye. The bony orbits, eyelids, tarsal glands and lacrimal apparatus protect the eyes. The inner eyelid is a thin membrane known as the palpebral conjunctiva. The conjunctiva connects the eyelids to the eyeball. At the connection with the eyeball, a thin layer called the ocular conjunctiva forms a continuous layer on the external and anterior surface of the eye (except for the corneal region), superior to the white part of the eye, the sclera. The conjunctiva contains blood vessels and nerves that support the avascular sclera and detect foreign particles entering the eye.
The lacrimal apparatus is responsible for the production and drainage of lacrimal fluid (tears). Tears reduce friction of the eyelids, continuously clean the anterior surface of the eye, and prevent bacterial infection through the action of lysozyme. Tears are produced by the lacrimal gland, located within the superolateral depression of each orbit. The superior and inferior lacrimal puncta are small openings to drain the lacrimal fluid into channels called the superior and inferior lacrimal canaliculi. From there, the lacrimal fluid enters the lacrimal sac and drains into the nasolacrimal duct that delivers it into the nasal cavity where it mixes with the mucus.
There are six extraocular muscles innervated by the abducens nerve (CN VI), trochlear nerve (CN IV) and oculomotor nerve (CN III). The superior rectus elevates the eye, the medial rectus adducts the eye, the inferior rectus depresses the eye, the lateral rectus abducts the eye. The superior oblique abducts, depresses, and internally rotates (intorsion) the eye. The inferior oblique abducts, elevates, and externally rotates (extorsion) the eye.
The eye walls are made by three layers of tissue called tunics. The fibrous tunic includes the white sclera and the clear cornea. The vascular tunic composed of the choroid, ciliary body attached to the lens by suspensory ligaments and iris (colored part of the eye). The iris is composed of pigmented layers and two layers of smooth muscle called the sphincter pupillae and dilator pupillae, that open and close the pupil. The neural tunic (or retina) includes a pigmented layer and a neural layer that contains the photoreceptors. The eye is divided in two cavities: the anterior cavity between the cornea and lens, filled with aqueous humor, and the posterior cavity behind the lens, filled with vitreous humor. The retina is composed of several layers of cells, connected to each other, that support vision transduction. The photoreceptors are rods for light vision and cones for color vision. Retinal ganglion cells have axons that collect at the optic disc and leave the eye as the optic nerve (CN II). At the exact center of the retina is a small area known as the macula lutea with a depression in the middle called the fovea. At the fovea, the retina lacks the supporting cells and blood vessels, and only contains cones. Therefore, visual acuity, or the sharpness of vision, is greatest at the fovea.
The visual pathway segregates information from the two eyes so that one half of the visual field projects to the other side of the brain. Within visual cortical areas, the perception of the stimuli and their location is passed along two streams, one ventral and one dorsal. The ventral visual stream connects to structures in the temporal lobe that are important for long-term memory formation. The dorsal visual stream interacts with the somatosensory cortex in the parietal lobe, and together they can influence the activity in the frontal lobe to generate movements of the body in relation to visual information.
Review Questions
Query \(\PageIndex{1}\)
Critical Thinking Questions
Query \(\PageIndex{2}\)
Glossary
Query \(\PageIndex{3}\)
Contributors and Attributions
OpenStax Anatomy & Physiology (CC BY 4.0). Access for free at https://openstax.org/books/anatomy-and-physiology